Wattenberg field
Wattenberg field
Giant Fields of the Decade: 2010-2020 | |
![]() | |
Series | Memoir |
---|---|
Chapter | Field Growth in the Supergiant Wattenberg Field, Denver Basin, Colorado, USA |
Author | Stephen A. Sonnenberg |
Link | Web page |
Field growth is a phenomenon where the estimates of known recovery tend to increase systematically over time. Generally, field growth is associated with the following: (1) boundaries of proved areas are extended by drilling; (2) new pay zones, pools, and reservoirs are found by drilling or recompletions; (3) infill wells or stimulation procedures; (4) improved drilling and completion costs; (5) and secondary or tertiary recovery. Items 1 through 4 occurred at Wattenberg, and item 5 is currently being tested. Unconventional fields experience field growth just like conventional fields. The overall economics of conventional and unconventional systems benefit from the field growth phenomenon.
The giant Wattenberg Field of Colorado was discovered in 1970 by Amoco Production Company with initial production from the Lower Cretaceous Muddy (J) Sandstone. Wattenberg straddles the Denver Basin synclinal axis and is regarded as a basin-center stratigraphic petroleum accumulation covering approximately 2600 mi2. Subsequent to discovery, the number of producing layers and production grew to include five additional formations (Dakota Plainview, Codell Sandstone, Niobrara Formation, Terry and Hygiene sandstone members of the Pierre Shale). The Terry and Hygiene were first produced in 1971, the Codell in 1981, the Niobrara in 1985, and the Dakota Plainview in 1998. Production occurs from approximately 4000 to 8500 ft below the surface across the field. Reservoir quality in the various horizons is generally poor, which mandates hydraulic fracture stimulation for economic production.
The addition of multiple productive horizons in the field area has significantly added to the total reserve number. The field is ranked by the U.S. Energy Information Administration (EIA) based on reserves as the fourth-largest oil field and the ninth-largest gas field in the United States.
Original reserves were estimated to be 1.1 TCFG for the Muddy (J) Sandstone. Fifty years after discovery, the field is currently at peak production because of recent horizontal drilling activity in the Codell and Niobrara with cumulative production to date at 1060 MMBO and 9.4 TCFG from more than 40,000 wells. Estimated ultimate recovery is 1100 MMBO and 9.7 TCFG. This represents reserve growth of over 1000% from discovery.
Hydrocarbon source rocks in Wattenberg are the Skull Creek Shale, Graneros Shale, Greenhorn Limestone, Carlile Formation, Niobrara Formation, and Sharon Springs Member of the Pierre Shale. Total source rock thickness in Wattenberg is 200 to 250 ft (>2 wt.% TOC). The source beds are dominantly Type II with some Type III kerogen present.
The Wattenberg area is a “hot spot” or positive temperature anomaly. This is an important reason the area is so prolific. Temperature gradients range from 1.6°F to 1.8°F/100 ft on the edges of the field to about 2.8°F to 2.9°F/100 ft in high gas-oil ratio (GOR) areas. The temperature anomaly is related to where the Colorado Mineral Belt intersects the Denver Basin. The mineral belt is a northeast trending zone across Colorado of Late Cretaceous to early Tertiary mineralization. The mineralization is associated with high geothermal gradients and hot fluids. The high heat flow and hydrocarbon generation contribute to abnormal pressure in parts of the field.
Introduction[edit]
Wattenberg Field is a supergiant oil and gas field located in the synclinal part of the Denver Basin (Figure 1). The field was discovered by Amoco Production Company in 1970 with completions in the Lower Cretaceous Muddy (J) Sandstone. Initial reserves for the Muddy (J) Sandstone were estimated to be 1.1 TCFG[1]. After the Muddy (J) development commenced, additional pays were discovered in the Terry and Hygiene sandstones (1972), Codell Sandstone (1981), Niobrara Formation (1984), and Dakota Lytle Formation (1998). Each of these formations contributes to field growth for the field (Figure 2). Also occurring was infill drilling activities and commingling rule changes that encouraged additional completions. Starting in 2010, the field started seeing horizontal drilling in the Codell and Niobrara, which has dramatically increased production (Figure 3A, B).
Figure 2 Production map for Wattenberg Field with various producing horizons color coded. Modified from Ladd[2]; unpublished map created by John Wright and Robert Weimer. Note the original boundary of the field compared to current producing areas.
Figure 3 (A) Production curve for Wattenberg Field area (includes Spindle, Hambert, Aristocrat, LaPoudre fields). The field was discovered in 1970. Horizontal drilling commenced in 2010. The addition of new horizons contributes to field growth. (B) Production curve for horizontal wells for Wattenberg Field. Modern horizontal drilling with multistage completions started in 2010. This production is included in Figure 3A. The new drilling and completion technologies contribute to field growth.
The greater Wattenberg area (GWA) covers approximately 2600 mi2. Production occurs from approximately 4000 to 8500 ft across the field. Cumulative production from the field is currently 1060 MMBO and 9.4 TCFG from more than 40,000 wells. Estimated ultimate recovery (EUR) is 1100 MMBO and 9.7 TCFG. The field is currently at peak production because of recent horizontal drilling activity in the Codell and Niobrara. The field growth observed in the field greatly exceeds original estimates of gas in place. The addition of multiple productive horizons in the field area has significantly added to the total reserve number. Other critical factors contributing to field growth are step out and infill drilling, technology advances (drilling, stimulation, and completion), horizontal drilling, and recompletions through time. The operators in the field have also changed through time, which probably also contributed to field growth by bringing in new ideas for production enhancements. The field is ranked by the Energy Information Administration (EIA) based on reserves as the fourth largest oil field and the ninth largest gas field in the United States[3]. The field is the largest field in Colorado based on cumulative production.
The Wattenberg field is regarded as a basin-center type of accumulation. Characteristics of a basin-center accumulation include regionally pervasive accumulations that are hydrocarbon saturated, commonly lack down-dip water contact, have low permeability reservoirs, and have low recovery factors. Wattenberg meets all these criteria. Basin-center accumulations are one of the more economically important petroleum systems in the world. In general, they will produce 10%–50% of the total annual oil and gas production in a region. Basin-center accumulations are commonly referred to resource plays in that they have somewhat predictable and repeatable results and economics. The low recovery factors and low permeability reservoirs mandate advanced completion and stimulation techniques (e.g., horizontal drilling and multistage hydraulic fracture stimulation).
The Wattenberg area is a “hot spot” or positive temperature anomaly ((Figure 4). This is an important reason the area is so prolific. Temperature gradients range from 1.6°F to 1.8°F/100 ft on the edges of the field to about 2.8°F–2.9°F/100ft in high GOR areas.[4] The temperature anomaly is related to where the Colorado Mineral Belt intersects the Denver Basin.[5] The mineral belt is a northeast trending zone across Colorado of Late Cretaceous to early Tertiary mineralization. The mineralization is associated with high geothermal gradients and hot fluids. The high heat flow and hydrocarbon generation contribute to abnormal pressure in parts of the field.
Previous work[edit]
The Wattenberg Field has a long development history and also publication history. Previous studies on Wattenberg have been conducted by Matuszczak[1]; Weimer[7][8][9]; Weimer and Sonnenberg[10][11]; Ladd[2]; Sonnenberg[12][13][14][15]; Thul and Sonnenberg[5]; and others.
The Muddy (J) Sandstone reservoir has previously been interpreted as a delta front and valley-fill deposits,[9] the Codell Sandstone as a shallow marine shelf sand[9][13], the Niobrara as deeper water chalks and marls[9][12], the Terry and Hygiene sandstones as shelf sands[9][16], and the Dakota Plainview as fluvial[9]. The reservoir quality of the various formations is generally low because of diagenesis in the basin-center setting.
Aims and objectives[edit]
The aim and objective of this chapter is to demonstrate the field growth history of the Wattenberg Field. Field growth shown mainly by the production curve shown in Figure 3A has been continuous through time. Reservoir quality in the various horizons is generally poor, which mandates hydraulic fracture stimulation for production. Field growth has greatly contributed to the economic significance of this field.
Both technological and geological factors influence production in resource [17]. Geological factors influencing productivity include reservoir thickness, reservoir quality, oil and gas saturations, hydrocarbon generation potential, maturity, overpressure, structure and lineaments, regional stress regime, mechanical stratigraphy, natural fractures, migration, and trapping mechanisms. Technological factors include well type, lateral length, number of hydraulic fracturing stages, proppant volume and type, proppant loading, fluid volume and type, fluid/proppant ratio, injection rate, treatment pressure, choke size, plug and perf versus sliding sleeves, and well spacing. All these factors influence production in Wattenberg Field and other unconventional accumulations.
Continuous accumulations[edit]
The Wattenberg Field is regarded as continuous type of hydrocarbon accumulation located in the basin center. The Wattenberg continuous accumulations have the following characteristics:
- Pervasive hydrocarbon saturated reservoirs
- No down-dip hydrocarbon/water contact
- Up-dip contact with regional water saturation
- Some water production
- Abnormally pressured (+ or −)
- Production independent of structural closure
- Low porosity and permeability reservoirs
- Sweet spots controlled by fractures and matrix
- Associated with mature source rocks that either are actively generating or have recently ceased generation
- Hydrocarbons of thermal origin
- The field boundary enlarged through time with step out drilling
Reservoirs that are pervasively hydrocarbon saturated in the Wattenberg area include the Muddy (J) Sandstone, Codell Member of the Carlile, and Niobrara formations. These reservoirs are not associated with down-dip water. The reservoirs are tight, generally lack water production, and require fracture stimulation to produce. The Muddy (J) is subnormally pressured, whereas the Codell and Niobrara are overpressured. Production occurs in the synclinal part of the Denver Basin and is independent of structural closure. The reservoirs are regarded as tight with low permeabilities (generally <0.1 md). Fractures are noted in cores and are thought to contribute to production. Associated source rocks include the Skull Creek Shale, Mowry Shale, Huntsman Shale, Graneros Shale, Greenhorn Formation, Carlile shales, and Niobrara Formation. The source rocks are thermally mature and in both the oil and gas windows in the field area. The field boundary of Wattenberg has enlarged over time in that it is a diffuse and not sharp boundary. Up-dip from Wattenberg are water-wet reservoirs suggesting the inverted system. Inverted systems have low reservoir properties compared to conventional reservoirs.
Wattenberg pre- and post-discovery[edit]
The Wattenberg area prediscovery is shown by Figure 5. The map illustrates pre-1970 wells in the GWA. The blue box outlines the area designated as GWA by the Colorado Oil and Gas Commission. Special field rules in this area allow infill drilling and the commingling of production from any Cretaceous reservoir.
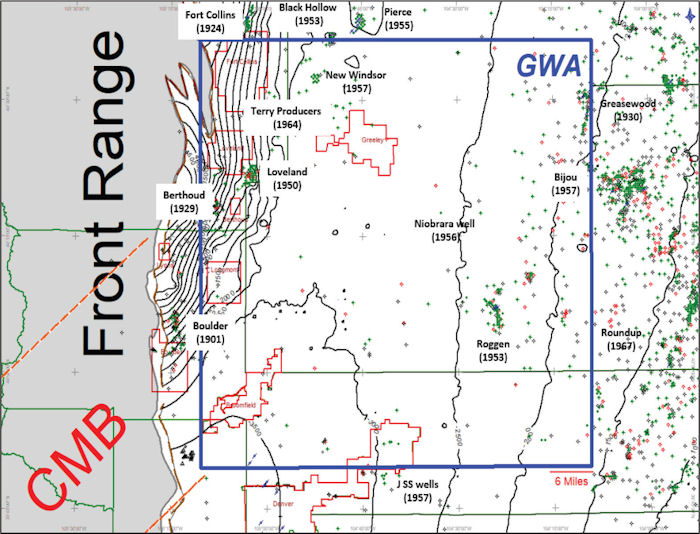
Clues to the existence of Wattenberg included the following: (1) all drill stem tests or cores taken in GWA indicated shows of oil or gas, (2) the Muddy (J) Sandstone in logs is remarkably similar across GWA and similar to reservoir properties of Cretaceous reservoirs of the San Juan Basin, (3) several old Muddy (J) wells had been completed in the southern area in 1957 (the Roundup gas field productive from the Muddy (J) was discovered in 1967 suggesting potential in the deep basin area), (4) shallow structural fields within or adjoining Wattenberg include Boulder (discovered in 1901 and productive horizons include Pierre Shale, Niobrara, and Codell); Berthoud Field (discovered in 1929 and productive from the Hygiene, Niobrara, Muddy (J) Sandstone, and Dakota Sandstone); Loveland Field (discovered in 1950 and productive from the Hygiene, Niobrara, Muddy (J), Dakota Sandstone, and Lyons Sandstone); Pierce, Black Hollow, and New Windsor fields (discovered in the 1950s and productive from the Lyons Sandstone); and Roggen Field (discovered in 1953 and productive from the Muddy (D) and Muddy (J) sandstones).
Amoco Production Company had an option on Union Pacific Railroad lands in the GWA and commenced drilling of wells in 1970[1]. Matuszczak[1] is the geologist credited with the discovery. He was convinced the area could be productive but also recognized that the wells would require fracture stimulation because of poor reservoir quality indicated on well logs and drill stem tests.
Figure 6 illustrates wells in the GWA assigned to the Wattenberg Field. The GWA includes other fields, but only wells assigned to Wattenberg will be tabulated for production data. Many companies (± 40) have been involved in the development of Wattenberg Field over the past 50 years. The following list includes some of the evolution of operators over time:
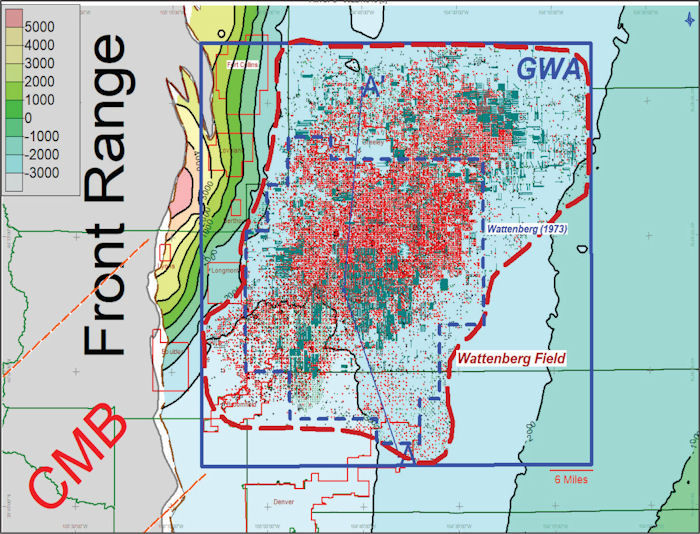
- Early (1970s): Amoco and Vessels
- Codell (1981): Energy Oil
- Price collapse mid-1980s
- Price recovery and tight gas sand credits late 1980s
- Late 1980s: Gerrity, Snyder, Martin Exploration, Prima, Basin, HS Resources
- 1991: Amoco farm out Codell to HS Resources and Snyder
- Amoco sells Wattenberg to HS Resources in 1997; Patina forms in 1996 (consolidation of Snyder and Gerrity properties); Noble acquires Patina in 2005
The top 10 current companies are Anadarko (now Occidental), Noble (now Chevron), PDC, Crestone Peak, Synergy, Extraction, Bonanza Creek, Great Western, Bill Barrett, KP Kauffman, and others.
Well spacing in GWA has evolved or changed significantly over the past 50 years. The following lists some of the more important changes in the spacing history:
- 1970: 320-acre units for drilling and spacing of J Sand
- 1979: Additional J Sand well allowed per 320-acre unit
- 1983: Codell spaced on 80 acres
- 1984, 1985: Niobrara added to Codell spacing order
- 1991: J Sand wells can be recompleted to Codell and Niobrara (C-N) and commingling of all downhole zones allowed
- 1998: Rule 318A allows five wells per quarter section in GWA for all Cretaceous age formations (81 townships)
- 2005: Rule 318A modified to allow for section line and quarter section line wells (~20 acre spacing 27 townships)
Reservoirs[edit]
Most of the production from Wattenberg comes from Cretaceous reservoirs (Dakota, Muddy (J) Sandstone, Codell Sandstone, Niobrara Formation, Hygiene Sandstone, and Terry Sandstone). Figure 7 is a north-south stratigraphic cross section through the central Wattenberg area. Location of the section is shown in Figure 8. The Wattenberg High paleostructure trends west to east across GWA and curves to the northeast in the eastern part of the mapped area. This feature was discussed by Weimer and Sonnenberg[10]. This Wattenberg High is well illustrated on Figures 7 and Figures 8. The thin area shown in Figure 8 is interpreted to be because of erosional truncation and onlap of units in the upper Niobrara.
Figure 7 South to north stratigraphic cross section through Wattenberg Field. Section is datumed on top of the Niobrara Formation. Dramatic thinning of the Niobrara occurs as a result of erosional truncation and onlap onto a paleostructural feature known as the Wattenberg High. Location of the cross section is shown in Figure 8
Figure 8 Isopach map of J Sandstone to top of Niobrara interval. The Wattenberg High paleostructure trends west to east across Wattenberg and curves to the northeast in the eastern portion of the mapped area. CMB is an abbreviation for the Colorado Mineral Belt. GWA = Greater Wattenberg Area. Contour interval 50 ft.
The reservoirs discussed in this chapter are Plainview/Lytle (Dakota) sandstones, Muddy (J) Sandstone, Codell Sandstone, Niobrara Formation, and Terry and Hygiene sandstones. The Terry and Hygiene sandstones (shallower Upper Cretaceous sandstones) production is generally assigned to the Spindle, Hambert, Aristocrat, LaPoudre, and Wattenberg fields and will be discussed later in this chapter.
Plainview/Lytle Sandstones[edit]
The Plainview and Lytle sandstones (transgressive shallow marine and fluvial, respectively) have been described in the outcrop west of Wattenberg Field by MacKenzie[18], Weimer[9], and others. Figure 9 illustrates that this interval ranges in thickness from 100 to 140 ft in the mapped area. The Lytle contains interbedded sandstone and conglomeratic sandstone. The Lytle is 50–100 ft thick in the outcrop and consists of 60%–80% sandstone and 20%–40% mudstone[18]. Cross stratification is common in the sandstone beds. Mudstones are greenish gray to maroon. The sandstones and mudstones do not contain tracks, trails, or burrows. The basal contact with the Morrison is a scour surface and is placed at a lower thick sandstone. The thick sandstones are interpreted as nonmarine channel sands in a large alluvial plain environment. Porosities on logs range from 8% to 12%.
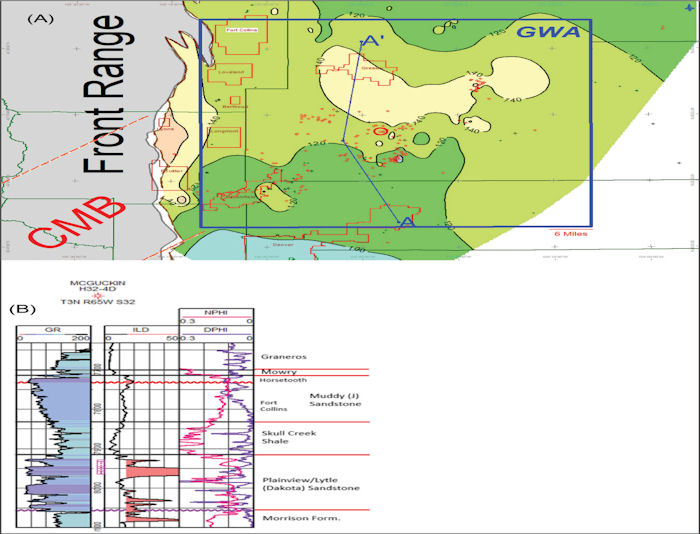
The top of the Lytle is a transgressive disconformity. The Plainview consists of very fine- to fine-grained sandstones and clay matrix. Ripple stratification and burrowing is common. The upper contact with the Skull Creek Shale is gradational into shale. The Plainview is interpreted to be a transgressive shallow-marine deposit. Porosities on logs are less than 10%.
Production from the Dakota (Plainview/Lytle) in the GWA dates back to 1974. Cumulative production is 2.0 MMBO, 87 BCFG, and 1.7 MMBW (million barrels of water) from approximately 180 wells. This production is not economic for stand-alone wells but could help total economics in commingled producing wells.
Muddy (J) Sandstone[edit]
The Muddy (J) Sandstone ranges in thickness from 40 to 150 ft across Wattenberg Field and consists of two members: an older Fort Collins Member and a younger Horsetooth Member[10][11][19][20] (Figures 7, 10, 11, 12). The Fort Collins consists of very fine- to fine-grained sandstone containing marine trace fossils and is interpreted to be a delta front deposit. The Horsetooth consists of fine- to medium-grained, well-sorted, cross-stratified sandstone. This sandstone is interpreted to have been deposited as part of a valley-fill deposit of fresh to brackish water origin. The sandstones contain carbonized wood fragments and are intercalated with siltstone and mudstone. The valley-fill model is shown in Figure 13. The sequence stratigraphy of the Muddy (J) Sandstone reservoir in Wattenberg field was discussed in detail by Weimer and Sonnenberg[11]. Regional distribution of the Muddy (J) and valley-fill systems have been published by Weimer and Sonnenberg[11], Dolson et al.[21], and others.
Figure 10 Type log for Wattenberg Field. Curves shown are gamma ray, spontaneous potential, resistivity, and neutron/density. Black bar in depth track indicates cored interval. Fort Collins Member designated F.C.M., and Horsetooth Member designated H.M. Note perforated interval 8160–8173 ft and associated neutron-density crossover gas effect.
Figure 11 Cored photographs for the Muddy (J) Sandstone from the Amoco #1 Rocky Mountain Fuel Well (Sec. 8, T1N-R67W). Log of cored interval shown in Figure 9. Muddy (J) interval is subdivided into Fort Collins and Horsetooth members. LDF = lower delta front; UDF = upper delta front; LSE = lowstand surface of erosion; TSE = transgressive surface of erosion. The well was perforated in the upper delta front, the main Muddy (J) pay in Wattenberg Field.
Figure 12 Isopach map of Muddy (J) Sandstone interval. The Muddy (J) Sandstone ranges in thickness from 160 ft to less than 60 ft across the mapped area. The thickest area is to the northeast, and thinnest area is to the west. See Figure 7 for cross-section A-A’. Contour interval 20 ft.
Figure Figure 13 Valley-fill model for the Muddy (J) Sandstone (from Weimer and Sonnenberg[10]). (A) Deposition of Fort Collins Member, wave-dominated deltaic progradation overlying Skull Creek Shale, (B) truncation of older marine and deltaic deposits with sea level lowering, and (C) sea level rise and back filling of valley networks with fluvial and marine strata (Horsetooth Member).
The Muddy (J) Sandstone ranges in thickness from 60 to 160 ft across the mapped area; the reservoir is productive over an area of 800,000 acres at depths of 7400–8500 ft (Figure 12). Reservoir parameters are as follows: pay thickness 10–50 ft, porosity range 8%–12%, permeability range 0.1–0.05 md, reservoir temperature 260°F, reservoir pressure 2750 psig, average water saturation 44%, and initial potentials 100–3575 MCFGPD. Initial reserves were estimated by Matuszczak[1] to be 1.1 TCFG from 283,000 acres. Updated reserves are 3.1 TCFG from 800,000 acres. Original well spacing was 320 acres, and current spacing is approximately 20 acres.
Most of the production comes from the Fort Collins Member (fine-grained delta front sandstone), which ranges in thickness from less than 25 ft to more than 100 ft (Figures 11, (12, 13, 14, 15). Thin areas in the Fort Collins are caused mainly from erosional incisement by Horsetooth valleys. The main pay section in the Fort Collins varies from 10 to 20 ft in thickness. The Fort Collins sandstones consist of approximately 80% quartz, 10% argillaceous matrix, 5% rock fragments, and 5% feldspar. Clay is the main matrix material, which is both detrital and diagenetic. The sandstones are bioturbated, and the amount of bioturbation decreases vertically in abundance. Higher energy conditions in the upper Fort Collins winnow out the clay fraction. Trace fossils change vertically from largely deposit feeders (lower Fort Collins) to suspension-feeding organisms (upper Fort Collins). Porosity is mainly intergranular with minor microporosity found in the matrix. The most important diagenetic event in the Fort Collins is formation of quartz overgrowths. The percentage of quartz overgrowths decreases as the clay content increases. Pores are triangular in shape because of the quartz overgrowths. Figure 15 illustrates location of wells that have cumulative production in excess of 500 MMCFG. The production sweet spot coincides with an isopach thick in the Fort Collins Member. Also important to production is the Wattenberg temperature anomaly, which is represented by the vitrinite reflectance contours.
Figure 14 Isopach map of the Fort Collins Member of the Muddy (J) Sandstone. The Fort Collins ranges in thickness from 25 to 100 ft across the mapped area. The thickest area is located south of Greeley. See Figure 7 for cross-section A-A’. The best production occurs between Broomfield and Greeley where the total Fort Collins interval is 75–100 ft thick. GWA = Greater Wattenberg Area. Contour interval 25 ft.]]
Figure 15 Isopach map of the Fort Collins Member of the Muddy (J) Sandstone. Contour interval is 25 ft. Also shown are Muddy (J) wells, which have cumulative production greater than 500 MMCFG. The thickness in Fort Collins is the sweet spot area for production. Also shown are Ro values for shales above the Muddy (J). Vitrinite reflectance contours modified from Higley and Cox[22] and Smagala et al.[23]. GWA = Greater Wattenberg Area. The best Muddy (J) production generally coincides with the highest maturity areas and thickest Fort Collins.
The Horsetooth Member ranges in thickness from less than 25 ft to more than 125 ft across Wattenberg Field (Figure 16). The sandstones are fine to medium grained, well sorted, and rounded and consist of 57%–80% quartz, 5%–10% rock fragments, and 5% feldspar. Porosity is intergranular with minor microporosity and intraparticle porosity. The Horsetooth Member is thickest in the northeast, southeast, and southwest parts of the mapped area. Diagenesis is similar to the Fort Collins Member with abundant silica cement along with smectite, chlorite, and kaolinite clay pore fillings. The clays can contribute to low-resistivity pay in the Horsetooth Member.
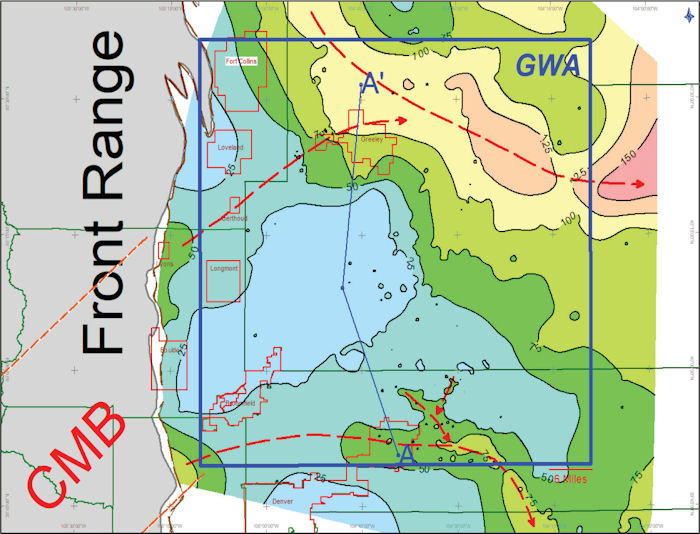
The best production in the Muddy (J) in Wattenberg comes from the Fort Collins Member (Figures 15, 17). The Horsetooth valley fills are also productive in Wattenberg but in isolated areas (largely because of intense diagenesis). In areas outside of Wattenberg, the Horsetooth is the more important Muddy (J) reservoir. Figures 14] and 16 show wells that have cumulative production of greater than 500 MMCFG to date. These wells are located along the basin synclinal axis and mainly where the Fort Collins Member is the thickest.
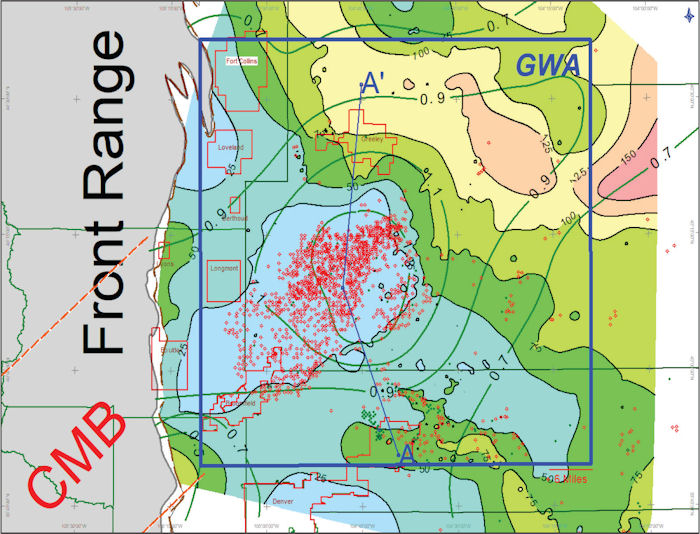
The thickest area of Fort Collins appears to be associated with the Wattenberg High. Horsetooth valley-fill systems occur surrounding the area of thick Fort Collins. The valley-fill systems appear to be associated with topographic low areas (Figure 13).
Codell Sandstone[edit]
The Upper Cretaceous Codell is a sandstone sequence of rocks that occurs between the main carbonate-producing episodes of the Cretaceous, namely the Niobrara and Greenhorn formations (Figure 7). The Niobrara and Greenhorn formations consist of chalks and marls and are thus deep-water carbonate deposits. The Codell probably represents a sea level lowering in between two of the highest sea levels during the Cretaceous. The interpreted depositional environment for the Codell Sandstone is as a shelf sand or shoreface[24].
The Codell Sandstone is a major pay in the giant Wattenberg Field of the Denver Basin. The mid-Turonian Codell Sandstone Member of the Carlile Formation has long been a focus as an exploration and development target in the Denver Basin. The first oil production from the Codell dates back to 1901 and the Boulder Oil Field development<refname=HigCox />. Vertical well completions within the Wattenberg Field date back to 1981 and were hydraulic fracture stimulated. The drilling depths for the Codell in Wattenberg range from 4000 to 8200 ft. Although extensively drilled with vertical wells, the Codell is now being developed via horizontal wells in Wattenberg Field. The vertical wells also have a history of successful hydraulic refracturing[25]. New horizontal wells (2011 to present) with initial production of 100–700 BOPD (GOR ~10,000 cf/bbl) indicate substantial remaining reserves in the formation.
Geologic factors important for production include proximity to thermally mature source beds, thickness, geothermal gradients, pressure gradients, fault-bounded reservoir compartments, GORs, and sufficient reservoir quality (phi-h). The Codell in Wattenberg is characterized by low porosity (<12%) and permeability (<0.1 md). The Codell is 5–20 ft thick across the Wattenberg Field and has formation pressure gradients that range from 0.45 to 0.66 psi/ft. Geothermal gradients range from 1.8°F to 3°F/100 ft. The highest GORs in the field correspond to the highest geothermal gradients. The sandstone is very fine to fine grained and bioturbated. Thin (<1 ft thick) hummocky cross-stratified beds are present in the Codell. Depositional environment is interpreted to be a shallow marine shelf setting. Clay content within the pay interval is approximately 20% and consists of 40%–45% mixed layer illite-smectite, 30%–40% illite, 10%–30% chlorite, and up to 7% glauconite. The Codell reservoirs have low-contrast, low-resistivity pay zones.
The Codell Sandstone is a member of the Carlile Formation and is middle Turonian in age. The Carlile Shale consists in ascending order the Fairport Chalk, Blue Hill Shale, and Codell Sandstone members. The Codell was named by Bass in 1926[26] for exposures in a bluff of the Saline Valley, 5 mi south-southwest of Codell, Kansas. Glenister and Kauffman[27] date the Codell as late Middle Turonian and assigned a late Turonian age to the Juana Lopez (thin brown limestone calcarenite), which lies disconformably above the Codell. Weimer and Sonnenberg[24] documented an unconformity at the base of the Codell Sandstone in the Wattenberg area. The Blue Hill Shale Member of the Carlile is truncated in the Wattenberg area. The Codell unconformably overlies the Fairport Chalk Member of the Carlile Formation and is unconformably overlain by either the Juana Lopez or the Fort Hays Limestone Member of the Niobrara Formation.
The Codell in Wattenberg ranges in thickness from a wedge-edge to more than 30 ft ((Figure 18). The Codell thickens westward across the field. An isopach map of Codell Sandstone greater than 6% porosity is shown in Figure 19. Porosity thickness declines to the east and thickens to the west.
Figure 18 Isopach map of the Codell Sandstone; contour interval 10 ft. Codell ranges in thickness from 5 to 30 ft across the mapped area. Thickest area is to the west. Codell is a low-resistivity, low-contrast pay as shown on Figure 20. GWA = Greater Wattenberg Area. Blue dashed lines show area where Codell is productive. Red dashed lines show Codell overpressured area (>0.5 psi/ft). Contour interval 5 ft.
Figure 19 Isopach map of the Codell Sandstone, porosity greater than 6% using 2.68 matrix density; contour interval 5 ft. Porous Codell ranges in thickness from 5 to 25 ft across the mapped area. Thickest area is to the west. Codell pressure greater than 0.5 psi within orange dashed area. Synclinal axis at Codell level shown by dashed gray line. Outline of where Codell produces shown by dashed blue line. GWA = Greater Wattenberg Area.
The Codell Sandstone in Wattenberg is an impermeable, bioturbated, fine-grained marine shelf sandstone[24]. Occasional thin hummocky cross-stratified beds (1–2 ft thick) are present in cores and the outcrop sections of the Codell (Figures 20, 21). The Codell Sandstone in Wattenberg is poorly to moderately sorted, very fine- to fine-grained sublitharenites and subarkose. Slight to moderate compaction and ductile grain deformation is common. Partial dissolution of chert and mica has created minor amounts of secondary porosity. Cements include mixed layer illite/smectite, illite, chlorite, quartz overgrowths, and calcite. Detrital clay further limits reservoir quality. The Codell in Wattenberg is a classic low-resistivity, low-contrast pay (LRLC; Figures 7, 20).
Figure 20 (A) Type log Codell Sandstone, Wattenberg Field. Gamma ray, spontaneous potential, resistivity, neutron, and density curves shown. Codell is a low resistivity, low contrast pay. (B) X-ray diffraction data for the Dome Frank core[28].
Figure 21 Core photographs of Dome Frank Codell core. Two distinct facies are present in core: (1) bioturbated sandstone and (2) low-angle cross stratified (hummocky beds). Unconformities are present at the top and base of Codell. From USGS Core Research Center[28].
The Codell has very little gamma-ray response and very little spontaneous potential (SP) response. Abnormally high porosity readings occur on density logs as a result of gas saturation (Figures 7, 20). Current development activity in Wattenberg Field includes horizontal wells with multistage hydraulic fracturing. The low resistivity is because of clay content of the sandstones. Values of porosity and permeability are 14% and 0.05 md for Niobrara/Codell wells in Wattenberg Field[22][29]. Based on core testing, the Codell Sandstone in Wattenberg Field has an average porosity and permeability of 14% and 0.1 md, respectively<refname=HigCox />.
The best Codell production coincides with an area of high heat flow in the Wattenberg Field that is centered south of Greeley and north of Denver (Figures 18, 19). The high heat area is reflected on temperature maps and by vitrinite reflectance data. The production “sweet spot” in the Codell coincides largely with high pressures and high heat flow (Figure 19).
Abnormal pressure in the Codell has been previously mapped by Birmingham et al.[25] (Figure 19). Abnormal pressure is present in the Greeley and south Greeley areas. Gradients as high as 0.65 psi/ft are observed in the Greeley area. The areas of highest pressure coincide with areas of highest production. The abnormal pressure area coincides with the high GOR area. The abnormal pressure is related to hydrocarbon generation[30].
Temperature, pressure, and reservoir net pay all contribute to the production sweet spot in the Codell Sandstone. Hydraulic fracturing of the Codell Sandstone is essential for economic production. The hydraulic fractures connect large areas of microporosity and microfractures to the wellbore. Many Codell wells effectively drain 15–30 acres after hydraulic fracture stimulation[25].
Completions vary for Codell/Niobrara vertical wells. Completions range from injecting 70,000 gallons of fluid and 200,000 pounds of sand to 180,000 gallons of fluid and 575,000 pounds of sand<refname=HigCox />. Solution gas and retrograde condensate behavior are reservoir drive mechanisms.
Codell refracturing (refracs) treatments are common and very successful in many areas of Wattenberg Field, but the high GOR areas show the best results or [25]. Refracturing may promote longer lateral extension hydraulic fractures than the original treatment. Original fracture wings are approximately 300 ft and extensions of 300–400 ft occur with refracturing. An incremental 25,000 BOE per well is common with the refracs. Horizontal drilling and multistage hydraulic fracturing is now being used in Wattenberg Field. The new horizontal wells are encountering new reserves from undrained areas. The Codell reservoir is productive over an area of 995,000 acres at depths of 6200–7900 ft. Reservoir parameters are as follows: gross pay thickness 10–30 ft, net pay 10–20 ft, porosity range 6%–12%, permeability range 0.1–0.005 md, reservoir temperature 260°F, reservoir pressure 3550–4615 psig, average water saturation 40%–50%, initial potentials in vertical wells ranges from 50 to 100 BOPD, and initial potentials in horizontal wells are greater than 500 BOPD. Original vertical well spacing was 80 acres, which was reduced to 20-acre spacing with down-spacing rules.
Niobrara Formation[edit]
The Upper Cretaceous Niobrara (Coniacian–Campanian; ~81–89.5 Ma) was deposited in a foreland basin setting in the Western Interior Cretaceous (WIC) Seaway of North America during a time of a major marine transgression[31][32]. This major transgression probably represents the maximum sea level highstand during the Cretaceous and may contain the best source rocks in the Cretaceous. The present-day basins in the Rocky Mountain region are formed during the Late Cretaceous to Early Tertiary Laramide orogeny.
The WIC Basin was an asymmetric foreland basin with the thickest strata being deposited along the western margin of the basin. The WIC Basin is a complex foreland basin that developed between mid to late Jurassic to Late Cretaceous time[33][34]. The basin was bordered by mountainous areas to the west (zone of plutonism, volcanism, and thrusting that formed the Cordilleran thrust belt) and a broad stable cratonic zone to the east. The foreland basin subsided in response to thrust and synorogenic sediment loading and pulses of rapid subduction and shallow mantle flow.
The stratigraphy of the Niobrara has been described by many authors[31][32][33]/>[35][36][37][38][39][40][41][42]. Source rock geochemistry of the Niobrara was described by Clayton and Swetland[43], Meissner et al. [30], and Weimer[9].
The Niobrara represents one of the two most widespread marine invasions and the last great carbonate-producing episode of the WIC Basin[33][40][41]. The Greenhorn interval is the other time of widespread marine invasion and carbonate production. The dominant lithologies of the Niobrara Formation are limestones (chalks) and interbedded marls (Figure 7). The chalk-marl cycles are interpreted to represent changes from normal to brackish water salinities possibly related to regional paleo-climatic factors or sea level fluctuations. The chalk lithologies are thought to represent deposition in normal to near-normal marine salinities having a well-mixed water column and well-oxygenated bottom waters. The chalks reflect influx of warm Gulfian currents into the WIC seaway during relatively high sea levels. The chalk/limestone facies is composed of coccolith-rich fecal pellets probably derived from pelagic copepods, inoceramid and oyster shell fragments, planktonic foraminifer tests, micrite, clay, and quartz silt[44]. The interbedded shale/marl cycles are interpreted to be caused by an increase in fresh water runoff caused by increased rainfall, which may be related to climatic warming. The fresh water runoff creates a brackish water cap and salinity stratification. Vertical mixing of the water column is inhibited causing anoxic conditions in the bottom waters. This enhances preservation of organic material and results in organic-rich source rocks. The decrease in water salinities is also suggested by oxygen isotopic values[40]. The shalier intervals may reflect lower sea levels and greater influx of clastic material from the west. The chalks have previously been interpreted to represent higher sea levels during Niobrara time[33].
The Niobrara consists of four limestone (chalk) units and three intervening marl intervals (Figures 7, 22, 23, 24). The lower limestone is named the Fort Hays, and the overlying units are grouped together as the Smoky Hill Member. The chalk units are referred to in descending order as the A, B, C, and Fort Hays. Erosional unconformities exist at the top and base of the Niobrara. The upper unconformity removes the upper chalk bed in some areas of the Wattenberg Field. The B and C chalks are the main focus of horizontal drilling by operators in the field.
Figure 23 Isopach Niobrara Formation; contour interval 50 ft. Niobrara ranges in thickness from 250 ft to more than 400 ft in mapped area. Ro contours modified from Smagala et al. .[23] and Higley and Cox<refname=HigCox />. Outline of where Niobrara produces shown by dashed orange line. Note coincidence of isopach thin area with Ro anomaly. GWA = Greater Wattenberg Area.
Figure 24 Type log for Niobrara Formation. Well was cored in the B and C chalk beds. Chalks generally have low gamma ray readings, high resistivity, and porosity development. This well was completed in the Codell Sandstone. Reservoirs in the Niobrara are the A, B, C, and Fort Hays chalk intervals. Source beds for the Niobrara include the Sharon Springs, Niobrara chalks, and also A marl, B marl, and C marl. The D marl is organic lean in Wattenberg area. The chalks have been targeted with vertical wells from 1981 through 2010. The play has now become a horizontal play targeting chalks and marls from 2010 to the present.
The Niobrara ranges in thickness from 300 to 400 ft across the field area (Figures 22, 23). The thickness changes in the Niobrara were described by Weimer and Sonnenberg[10]. The west to east thin area shown on Figure 22 was thought to be caused by a paleostructural feature of similar orientation (i.e., basement fault block). The thinning is due in part to erosional truncation of the A chalk unit over the broad east–west trending area. Figure 7 is a north–south stratigraphic cross section datumed on top of the Niobrara. Thickness changes in individual Niobrara chalks and marls units in the past have been noted by several authors and attributed to chalk bar formation (associated with bottom water currents), compensational stacking, paleotopography effects (low areas being isopach thicks and high areas being isopach thins), and submarine current erosion and scour. Numerous normal faults present within the Niobrara Formation create small circular isopach thins on the various isopach maps (bull’s-eyes). The normal faults create tremendous reservoir heterogeneity and potential problems for drillers trying to “stay in zone” in horizontal laterals. Figure 23 illustrates the location of the Wattenberg temperature anomaly superimposed on the Niobrara isopach map. The outline area of where the Niobrara produces is also shown on Figure 23.
A typical well log response for the Niobrara is shown in Figure 24. The chalk and marl intervals can be distinguished based on log response. In general, the chalks have a low gamma ray value than the marls. This is attributed to higher clay and total organic clay (TOC) in the marls compared to the chalks. The chalks tend to have higher resistivities than the marls because of the purer chalk lithology and presence of hydrocarbons. In addition, on neutron and density porosity logs, the curves track each other in the chalks and diverge because of increase in clay content in the marls.
One of the main producing chalk intervals in Wattenberg is the B chalk (Figures 24, 25). The B chalk has high resistivity (>50 ohms) and porosities on logs greater than 10%. The A and C chalks are also important producers in Wattenberg Field.
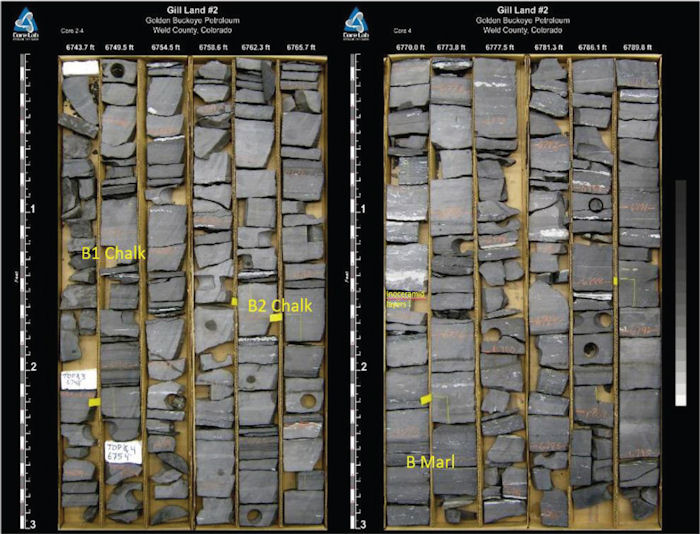
The Niobrara Formation reservoir is productive over an area of 1,145,000 acres at depths of 6300–7500 ft. Reservoir parameters are as follows: pay thickness 10–40 ft, porosity range 6%–10%, permeability range 0.05–0.0005 md, reservoir temperature 260°F, reservoir pressure 3400–3550 psig, average water saturation 40%–50%, initial potentials in vertical wells ranges from 50 to 100 BOPD, and initial potentials in horizontal wells are greater than 500 BOPD. Original vertical well spacing was 80 acres, which was reduced to 20 acre spacing with down spacing rules.
Terry and Hygiene sandstones[edit]
Production occurs in the Upper Cretaceous (Campanian) Hygiene and Terry sandstones in the Wattenberg area (Figures 26, 27, 28). Most of the production is from the Terry Sandstone. The sandstones are interpreted to be marine sandstones enclosed in finer-grained mudstones[16][45][46]. Sandstones are glauconitic, feldspathic litharenites. The depositional environment was interpreted by Porter[16] to be offshore marine bars (Figure 27). Coarsening upward sequences consist of three facies: bioturbated sandy mudstones; burrowed to nonburrowed, thin-bedded, rippled to cross-stratified sandstone; and fine- to medium-grained, cross-stratified sandstone. Sandstone facies are designated as sandy shelf, bar margin, and central bar. The marine bars in the Terry trend northwest-southeast. Bars are 2–4 miles wide and 8–16 miles long. The nature of the trap is stratigraphic. Source rocks are the Sharon Springs Member of the Pierre Shale and the Niobrara Formation.
Figure 26 Structure contour of Terry Sandstone marker bentonite bed. Contour interval 100 ft. Map shows Terry (green dots), Hygiene (red dots), and Terry/Hygiene commingled (pink dots) production. Vast majority of production comes from Terry Sandstone (green dots). Type log shown in this figure. Production curve shown in Figure 27. Field names from scout ticket reports. The Surrey, Spindle, and Singletree fields are now combined together into the Spindle Field. GWA = Greater Wattenberg Area. These fields are regarded as unconventional accumulations of stratigraphically trapped oil and gas. Fields are shallow pool accumulations in the Wattenberg Field.
Figure 27 (A) Type log for Terry and Hygiene sandstones, Wattenberg Field area. (B) Paleography for Upper Campanian. (C) Offshore shallow shelf model for sand bar development. Modified from Porter and Weimer[46].
Porosity averages 14%; permeability averages 2 md[16]. Producing depths are 4200–5200 ft. Reservoir thickness is 20–25 ft. Original field pressure is 1450 psi, drive mechanism is solution-gas drive, oil gravity is 42° API, and minimum water saturation is 30%. Average initial potential from wells is 125 BOPD. The Terry and Hygiene fields are regarded as unconventional stratigraphic traps of oil and gas. They are deemed unconventional because the reservoirs require fracture stimulation and the regional aspect of the hydrocarbon accumulations along with the general lack of produced water. The fracture stimulations are quite variable and generally range from 50,000 to 120,000 gallons fluid and 60,000 to 400,000 lb of sand.
Extensive secondary porosity was observed in thin sections and scanning electron microscope (SEM) analysis in both the Terry and Hygiene sandstones[46]. Leaching of calcite cement and calcite-replaced grains and matrix and dissolution of framework grains and pseudomatrix suggested the secondary porosity development.
Producing Hygiene and Terry fields include Spindle (combined Surrey, Spindle, and Singletree), Hambert, Aristrocrat, Wattenberg-Hygiene, Wattenberg-Terry, LaPoudre, and New Windsor (Figure 26). Total number of producing wells is approximately 2920 (2780 from the Terry, 140 from the Hygiene). Many of the Terry and Hygiene wells are assigned to the Wattenberg Field (1154) instead of having different field names. All the producing Terry and Hygiene wells could be considered as shallow pool accumulations in the Wattenberg Field. The oldest production from the Terry and Hygiene in the GWA is from the New Windsor field and dates to 1957. Spindle was discovered in 1972, Singletree in 1971, Surrey in 1972, Hambert in 1972, Aristocrat in 1978, and LaPoudre in 1981. The combined fields reached peak production in 1977 and have been steadily declining. Cumulative production from the Terry and Hygiene in GWA is 63 MMBO, 392 BCF gas, and 15 MMBW (Figure 28).
Source rocks[edit]
Source rocks for the Wattenberg area are the Skull Creek, Mowry, Huntsman, Graneros, Greenhorn, Carlile, and Niobrara formations[43][47] (Figures 1, 7). Note the SR (source rock) abbreviation on the stratigraphic column in Figure 1.
The source beds are all marine Type II kerogen with admixtures of Type III. This type of source bed can generate both oil and gas.
Clayton and Swetland[43] report the following ranges for organic carbon for the following source rocks: Skull Creek 0.8–2.5 wt.%, Mowry 0.8–3.6 wt.%, Huntsman 1.0–2.6 wt.%, Graneros 1.1–3.5 wt.%, Greenhorn 1.5 wt.%, and Niobrara 0.8–3.4 wt.%. Weimer[9] reports TOC values for the Graneros Shale section ranging from 1.06 to 4.4 wt.%. The Skull Creek, Mowry, Huntsman, and Graneros most likely source the Muddy (J) reservoirs. The Skull Creek may be the dominant source for Dakota Sandstone (deeper than the Muddy (J), includes Eldorado Springs and Plainview/Lytle). The Niobrara and Greenhorn most likely source the Codell Sandstone. The Niobrara is self-sourced, but contribution comes from the Sharon Springs. The Niobrara and Sharon Springs are the main source beds for the Terry and Hygiene sandstones.
Thermal maturity plays a significant role in production. Vitrinite reflectance contours were published for the Graneros, Huntsman, Mowry, and Skull Creek source rocks for the Denver Basin<refname=HigCox />. Vitrinite reflectance values of 0.9% Ro or greater define the Wattenberg Field. Values between 0.6 and 1.2 are generally indicative of the oil window. Values greater than 1.2 are indicative of reservoirs in the gas window. Values of 0.6% Ro represent the probable lower limit for oil generation.
Results and discussion[edit]
Field growth is a common phenomenon in oil and gas fields (Figure 3A, B). The field growth potential in unconventional plays is driven by economics, recovery factors, and technology. Field growth is a key component of unconventional resource plays. Field growth is a common phenomenon, which occurs in fields. The production and field growth in Wattenberg illustrates this concept. Fields grow for a variety of reasons, and the following list gives some of the explanations for the growth:
- Step outs (unconventional fields have fuzzy boundaries)
- Infill drilling or down spacing
- New horizons deeper or shallower reservoirs in a field (in the case of Wattenberg: Dakota Sandstone, Codell, Niobrara, Terry, and Hygiene)
- Improved economics (faster drilling, higher product prices, tax incentives, etc.)
- Improved geologic model suggesting undrained areas
- Improved reservoir modeling for history matching and production forecasting
- Improved technologies (horizontal drilling, completions)
- Fracture stimulation
- Recompletions (proved not producing horizons)
- Changing operator (a new company commonly has a different idea)
- Enhanced recovery (secondary or tertiary)
All these factors except for the last bullet apply to the field growth history in Wattenberg. Enhanced recovery is an option some operators are currently discussing. A production summary curve for Wattenberg, Spindle, Aristocrat, LaPoudre, and Hambert fields is shown in Figure 3A. The production is at record levels. The field growth associated with GWA demonstrates the resource power of unconventional fields.
The future[edit]
Future growth potential still exists within Wattenberg Field. Surface access may be the most limiting factor in Wattenberg growth because of tremendous urban growth in the field area. Balancing energy development and urban growth will continue to be the focus of legislation in the state of Colorado, and this creates an unknown future. All that said, new technology may be able to help settle some of the conflicts.
Conclusions[edit]
Wattenberg Field is perhaps the most significant field in the U.S. Rockies in terms of cumulative production, reserves, and field growth history. The reserves and production in the field have grown more than 1000% over the past 50 years. The field is a basin-center type of an accumulation with six producing horizons. The reservoirs are generally low permeability and require technology to produce (e.g., hydraulic fracture stimulation). Unconventional fields such as Wattenberg illustrate the importance of the field growth phenomenon associated with improving technology in part driven by favorable economics. Factors contributing to field growth include both geology and technology. The most important geological factors include having excellent source rocks in proximity to reservoirs. The source rocks and the temperature anomaly associated with the GWA create an ideal situation for source and reservoir beds. Paleostructure and the development of the Wattenberg High is also an important factor for the Wattenberg area. Overpressuring is reported in the Codell and Niobrara reservoirs[9]. Overpressure is interpreted to be caused by hydrocarbon generation. Reservoirs are generally tight (<0.1 md) and require hydraulic fracture stimulation. The drilling and completion technologies have improved dramatically over time resulting in faster drilling, completions, and economics.
References[edit]
- ↑ 1.0 1.1 1.2 1.3 1.4 Matuszczak, R. A., 1973, Wattenberg Field, Denver Basin, Colorado: The Mountain Geologist, v. 10, no. 3, p. 99–105.
- ↑ 2.0 2.1 Ladd, J. H., 2001, An overview and development history of the Wattenberg field, in D. Anderson, ed., Gas in the Rockies: Rocky Mountain Association of Geologists, Denver, Colorado, p. 29–42.
- ↑ EIA, 2015, Top 100 US oil and gas fields: US Energy Information Administration, accessed February 3, 2021, www.eia.gov.
- ↑ Meyer, H. J., and H. W. McGee, 1985, Oil and gas fields accompanied by geothermal anomalies in Rocky Mountain region: AAPG Bulletin, v. 69, no. 6, p. 933–945.
- ↑ 5.0 5.1 5.2 Thul, D. J., and S. A. Sonnenberg, 2018, Expression of the Colorado Mineral Belt in Upper Cretaceous Niobrara Formation source rock maturity data from the Denver Basin: The Mountain Geologist, v. 55, no. 1, p. 19–52.
- ↑ Thul, D. J., 2012, Niobrara source rock maturity in the Denver Basin: A study of differential heating and tectonics on petroleum prospectivity using programmed pyrolysis: Master’s thesis, Colorado School of Mines, Golden, Colorado, 157 p.
- ↑ Weimer, R. J., 1978, Influence of transcontinental arch on Cretaceous marine sedimentation: A Preliminary Report, in J. D. Pruit, and P. E. Coffin, eds., Energy resources of the Denver Basin: Rocky Mountain Association of Geologists, Denver, Colorado, p. 211–222.
- ↑ Weimer, R. J., 1980, Recurrent movement on basement faults, a tectonic style for Colorado and adjacent areas, in H. C. Kent, and Porter, K. W., eds., Colorado geology: Rocky Mountain Association of Geologists, Denver, Colorado, Guidebook, p. 23–35.
- ↑ 9.0 9.1 9.2 9.3 9.4 9.5 9.6 9.7 9.8 9.9 Weimer, R. J., 1996, Guide to the petroleum geology and Laramide Orogeny, Denver Basin and Front Range, Colorado: Colorado Geological Survey Bulletin, v. 51, 127 p.
- ↑ 10.0 10.1 10.2 10.3 10.4 Weimer, R. J., and S. A. Sonnenberg, 1982, Wattenberg Field, Paleostructure-stratigraphic trap, Denver Basin, Colorado: Oil and Gas Journal, v. 80, p. 204–210.
- ↑ 11.0 11.1 11.2 11.3 Weimer, R. J., and S. A. Sonnenberg, 1989, Sequence stratigraphic analysis of Muddy (J) Sandstone Reservoir, Wattenberg Field, Denver Basin, Colorado, in E. Coalson, ed., Sandstone reservoirs: Rocky Mountain Association of Geologists, Denver, Colorado, p. 197–220.
- ↑ 12.0 12.1 Sonnenberg, S. A., 2013, New reserves in an old field, the Niobrara Resource Play in the Wattenberg Field, Denver Basin, Colorado: Unconventional Resources Technology Conference, Paper 1562913, 13 p.
- ↑ 13.0 13.1 Sonnenberg, S. A., 2014, The Codell Sandstone Play of the Northern Denver Basin: Unconventional Resource Technology Conference, Paper 1917728, 11 p.
- ↑ Sonnenberg, S. A., 2015, Geologic factors controlling production in the Codell Sandstone, Wattenberg Field, Colorado: Unconventional Resource Technology Conference, Paper 2145312, 17 p.
- ↑ Sonnenberg, S. A., 2016, Stratigraphic architecture of the Niobrara Formation, Wattenberg Field Area, Colorado: Unconventional Resource Technology Conference, Paper 2435024, 15 p.
- ↑ 16.0 16.1 16.2 16.3 Porter, K. W., 1989, Structurally influenced stratigraphic and diagenetic trapping at Spindle field, Colorado, in E. Coalson, ed., Petrogenesis and petrophysics of selected sandstone reservoirs of the Rocky Mountain region: Rocky Mountain Association of Geologists, p. 255–264.
- ↑ Theloy, C., and S. A. Sonnenberg, 2013, Integrating geology and engineering: Implications for production in the Bakken play, Williston Basin: Unconventional Resource Technology Conference Paper 1596247, 12 p.
- ↑ 18.0 18.1 MacKenzie, D. B., 1971, Post-Lytle Dakota Group on west flank of Denver basin, Colorado: The Mountain Geologist, v. 8, p. 91–131.
- ↑ MacKenzie, D. B., 1965, Depositional environments of Muddy Sandstone, western Denver basin, Colorado: AAPG Bulletin, v. 49, p. 186–206.
- ↑ Weimer, R. J., S. A. Sonnenberg, G. B. C. Young, 1986, Wattenberg Field, Denver Basin, Colorado, ‘’in’’ C. W. Spencer, and R. F. Mast, eds., Geology of tight gas reservoirs: AAPG Studies in Geology 24, p. 143–164.
- ↑ Dolson, J. C., D. S. Muller, M. J. Evetts, and J. Stein, 1991, Regional paleotopographic trends and production, Muddy Sandstone (Lower Cretaceous), Central and Northern Rocky Mountains: AAPG Bulletin, v. 75, no. 3, p. 409–435.
- ↑ 22.0 22.1 22.2 Higley, D. K., and D. O. Cox, 2007, Oil and gas exploration and development along the front range in the Denver Basin of Colorado, Nebraska, and Wyoming, ‘’in’’ D. K. Higley, compiler, Petroleum systems and assessment of undiscovered oil and gas in the Denver Basin Province, Colorado, Kansas, Nebraska, South Dakota, and Wyoming—USGS Province 39: USGS Digital Data Series DDS–69–P, 41 p.
- ↑ 23.0 23.1 23.2 Smagala, T. M., C. A. Brown, and G. L. Nydegger, 1984, Log-derived indicator of thermal maturity, Niobrara Formation, Denver Basin, Colorado, Nebraska, Wyoming, in J. Woodward, F. F. Meissner, and J. C. Clayton, eds., Hydrocarbon source rocks of the greater Rocky Mountain region: RMAG, Denver, Colorado, p. 355–363.
- ↑ 24.0 24.1 24.2 Weimer, R. J., and S. A. Sonnenberg, 1983, Codell Sandstone, new exploration play, Denver Basin: Oil and Gas Journal, v. 81, no. 22, p. 119–125.
- ↑ 25.0 25.1 25.2 25.3 Birmingham, T. J., D. M. Lytle, and R. N. Sencenbaugh, 2001, Enhanced recovery from a tight gas sand through hydraulic refracturing: Codell Formation, Wattenberg Field, Colorado, ‘’in’’ D. S. Anderson, J. W. Robinson, J. E. Estes-Jackson, and E. B. Coalson, eds., Gas in the Rockies: Rocky Mountain Association of Geologists Special Publication, p. 101–116.
- ↑ Merriam, D. F., 1963, The geologic history of Kansas: Kansas Geological Survey Bulletin, v. 162.
- ↑ Glenister, L. M., and E. G. Kauffman, 1985, High resolution stratigraphy and depositional history of the Greenhorn regressive hemicyclothem, Rock Canyon Anticline, Pueblo, Colorado: SEPM Field Trip Guidebook 4, p. 170–183.
- ↑ 28.0 28.1 28.2 USGS Core Research Center, 2015, accessed February 4, 2021.
- ↑ Higley, D. K., D. O. Cox, and R. J. Weimer, 2003, Petroleum system and production characteristics of the Muddy (J) Sandstone (Lower Cretaceous) Wattenberg continuous gas field, Denver Basin, Colorado: AAPG Bulletin, v. 87, no. 1, p. 15–37.
- ↑ 30.0 30.1 Meissner, F. F., J. Woodward, and J. L. Clayton, 1984, Stratigraphic relationships and distribution of source rocks in the greater Rocky Mountain region, ‘’in’’ J. Woodward, F. F. Meissner, and J. L. Clayton, eds., Hydrocarbon source rocks of the greater Rocky Mountain region: Rocky Mountain Association of Geologists Guidebook, p. 1–34.
- ↑ 31.0 31.1 Longman, M. W., B. A. Luneau, and S. M. Landon, 1998, Nature and distribution of Niobrara lithologies in the Cretaceous Western Interior seaway of the Rocky Mountain Region: The Mountain Geologist, v. 35, no. 4, p. 137–170.
- ↑ 32.0 32.1 Landon, S. M., M. W. Longman, and B. A. Luneau, 2001, Hydrocarbon source rock potential of the Upper Cretaceous Niobrara Formation, Western Interior Seaway of the Rocky Mountain region: The Mountain Geologist, v. 38, no. 1, p. 1–18.
- ↑ 33.0 33.1 33.2 33.3 Kauffman, E. G., 1977, Geological and biological overview Western Interior Cretaceous Basin: The Mountain Geologist, v. 14., p. 75–99.
- ↑ Kauffman, E. G., 1985, Cretaceous evolution in the Western Interior Basin of the United States, ‘’in’’ L. M. Pratt, E. G. Kauffman, and F. B. Zelt, eds., Fine-grained deposits and biofacies of the Cretaceous Western Interior Seaway: Evidence of cyclic sedimentary processes: SEPM Field Trip 9, p. 90–99.
- ↑ Weimer, R. J., 1960, Upper Cretaceous Stratigraphy, Rocky Mountain Area: AAPG Bulletin, v. 44, p. 1–20.
- ↑ Hattin, D. E., and C. T. Siemers, 1978, Upper Cretaceous stratigraphy and depositional environments of western Kansas: Kansas Geological Survey Guidebook 3, 102 p. (reprinted 1987, with modifications).
- ↑ Hann, M. L., 1981, Petroleum potential of the Niobrara Formation in the Denver Basin—Colorado and Kansas, Master’s thesis, Colorado State University, Fort Collins, Colorado, 260 p.
- ↑ Sonnenberg, S. A., and R. J. Weimer, 1981, Tectonics, sedimentation, and petroleum potential northern Denver Basin Colorado, Wyoming, and Nebraska: Colorado School of Mines Quarterly, v. 7, no. 2, 45 p.
- ↑ Sonnenberg, S. A., and R. J. Weimer, 1993, Oil production from Niobrara Formation, Silo Field, Wyoming: Fracturing associated with a possible wrench fault system: The Mountain Geologist, v. 30, no. 2, p. 39–53.
- ↑ 40.0 40.1 40.2 Barlow, L. K., 1985, Event stratigraphy, paleoenvironments, and petroleum source rock potential of the Niobrara Formation (Cretaceous), northern Front Range, Colorado, Master’s thesis, University of Colorado, Boulder, Colorado, 288 p.
- ↑ 41.0 41.1 Barlow, L. K., 1986, An integrated geochemical and paleoecological approach to petroleum source rock evaluation, lower Niobrara Formation (Cretaceous), Lyons, Colorado: The Mountain Geologist, v. 23, p. 107–112.
- ↑ Rodriguez, T. E., 1985, High-resolution event stratigraphy and interpretation of the depositional environments of the upper Smoky Hill Member, Niobrara Formation of the northwest Denver Basin, M.S. thesis, University of Colorado, Boulder, Colorado, 197 p.
- ↑ 43.0 43.1 43.2 Clayton, J. L., and P. J. Swetland, 1980, Petroleum generation and migration in the Denver Basin: AAPG Bulletin, v. 64, p. 1613–1624.
- ↑ Lockridge, J. P., and P. A. Scholle, 1978, Niobrara gas in eastern Colorado and northwestern Kansas, ‘’in’’ J. D. Pruit, and P. E. Coffin, eds., Energy Resources of the Denver Basin: Rocky Mountain Association of Geologists Guidebook, p. 35–49.
- ↑ Moredock, D. E., and S. J. Williams, 1976, Upper Cretaceous Terry and Hygiene Sandstones, Singletree, Spindle, and Surrey Fields, Weld County, Colorado, ‘’in’’ R. C. Epis, and R. J. Weimer, eds., Studies in Colorado field geology: CSM Professional Contribution 8, p. 264–274.
- ↑ 46.0 46.1 46.2 Porter, K., and R. J. Weimer, 1982, Diagenetic sequence related to structural history and petroleum accumulation: Spindle field, Colorado: AAPG Bulletin, v. 66, no. 12, p. 2543–2560.
- ↑ Rice, D. D., and C. N. Threlkeld, 1983, Character and origin of natural gas from Upper Cretaceous Codell Sandstone, Denver Basin, Colorado: Mid-Cretaceous Codell Sandstone Member of Carlile Shale Eastern Colorado: The Rocky Mountain Section SEPM Spring Field Trip Guidebook, p. 96–100.