Mudstones
Sequence Stratigraphy: Applications to Fine-Grained Rocks | |
![]() | |
Series | Memoir |
---|---|
Chapter | The Importance of and Challenges in Studying Fine-Grained Sedimentary Rocks—Introduction and Overview |
Author | Kevin Bohacs & Remus Lazar |
Link | Web page |
Fine-grained sedimentary rocks (e.g., shale, mudrock, mudstone, claystone, chert, or chalk) are the most common rocks in the stratigraphic column. They preserve the best archives of Earth’s history for interpreting paleoclimate and paleo-oceanography; influence the flow of groundwater and hydrocarbons; serve as hydrocarbon source, reservoir, and seal; and host strata-bound resources. Advancing technology has provided access to the vast energy resources contained in these rocks—indeed, the production of “shale oil” and “shale gas” in the United States changed the energy outlook almost completely over the seven-year span of 2007–2014.
Key Attributes of Fine-Grained Sedimentary Rocks
Sequence-stratigraphic analyses rely on a firm understanding of the nature and character of the rocks under consideration to properly recognize the expression of stratigraphic surfaces and stratal packages in particular depositional systems. In addressing the nature of FGRs, there has been a significant variation in the usage of some of the terms used to describe these rocks. For a detailed discussion and comparison of different terms and classifications of FGRs, see Mudstone nomenclature, as well as Ingram[1]; Tourtelot[2]; Folk[3][4]; Picard[5]; Blatt et al.[6]; Lundegard and Samuels[7]; Potter et al.[8][9]; Spears[10]; Stow[11]; Macquaker and Adams[12]; Lazar et al.[13]/>[14][15]. Here, we recommend the use of “mudstone” as a class name for all FGRs based on their key attribute of grain size, in direct analogy with other sedimentary rock names such as “sandstone” and “limestone.” The following are the key attributes of mudstone to keep in mind throughout this book and in your interpretation of mudstone strata.
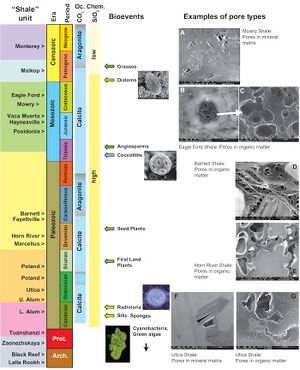
Mudstone, similar to sandstone or grainstone, is deposited layer by layer, in sequential order. Its present-day attributes are the result of the sediment history: provenance, weathering and erosion, transport, deposition, and diagenesis.
Mudstone is heterogeneous at sub-millimeter-to-decimeter scale vertically and at centimeter-to-kilometer scale laterally and contains a wide variety of sedimentary structures (e.g., Cluff[17]; Schieber[18],[19] Bohacs[20][21], O’Brien and Slatt[22]; Schieber[23][24][25]; Macquaker et al.[26][27]; Guthrie and Bohacs[28]; Lazar et al.[13][14][15]; Bohacs et al.[29]).
Practically all pelagic material is transported as near-bottom suspension or in bed load as aggregates, floccules, or pellets comprising individual clay- and silt-sized particles with significant amounts of biogenic binding material (e.g., Schieber et al.[30]; Macquaker et al.[31]).
Although transported and deposited as individual or composite particles, rapid consolidation and development of cohesiveness means that subsequent erosion tends to produce significantly larger clasts (as much as 10 cm or greater in diameter)[32][33][34][35].
Most fine-grained biogenic carbonate is advected along the bottom and acts as just another detrital component—very little accumulates in the place it was formed[36][37].
Mudstone composition varies as a function of varying contributions of (1) weathered fine-grained sediment delivered to the basin and (2) soft and hard organic material produced within the basin. This mix of fine-grained material undergoes biochemical transformations during and after mud deposition.
Pervasive silica or carbonate cements commonly form very early at low temperatures as a result of microbial consumption of organic matter and, thus, essentially record attributes of the depositional environment (e.g., Berner[38][39]; Macquaker and Taylor[40]). These cements are typically present just below stratigraphic surfaces (especially flooding surfaces or sequence boundaries). Hence, they can preserve the depositional profile and give it a distinctive well-log signature (e.g., Bohacs[20][21]; Macquaker and Jones[41]; Bohacs and Lazar[42][43]).
Mudstone is reactive because of the large surface area of its components and its biogenic content. These components tend to be unstable at both Earth’s surface and burial conditions, which can lead to extensive diagenetic effects on rock character.
Mudstone is relatively impermeable, so early diagenetic pathways are mostly set by starting (depositional) composition (unlike more highly permeable sandstone or coarse carbonate that could have been exposed to multiple pore volumes of reactants transported through them). Mudstone character changed significantly throughout the Phanerozoic because of the evolution of planktonic organisms (Figure 1; e.g., Niklas et al.[44]; Stanley and Hardie[45]; Markello et al.[46]; Bohacs et al.[16]; Carpenter and Keane[47]).
References
- ↑ Ingram, R. L., 1953, Fissility of mudrocks: Bulletin of Geological Society of America, v. 64, p. 869–878.
- ↑ Tourtelot, H. A., 1960, Origin and use of the word “shale”: American Journal of Science, v. 258A, p. 335–343.
- ↑ Folk, R. L., 1965, Petrology of sedimentary rocks, 1st edition: Austin, Texas, Hemphill’s, 159 p.
- ↑ Folk, R. L., 1968, Petrology of sedimentary rocks, 2nd edition: Austin, Texas, Hemphill’s, 170 p.
- ↑ Picard, D. M., 1971, Classification of fine-grained sedimentary rocks: Journal of Sedimentary Petrology, v. 41, p. 179–195.
- ↑ Blatt, H., G. Middleton, and R. Murray, 1980, Origin of sedimentary rocks: Englewood Cliffs, New Jersey, Prentice-Hall, Inc., 782 p.
- ↑ Lundegard, P. D., and N. D. Samuels, 1980, Field classification of fine-grained sedimentary rocks: Journal of Sedimentary Petrology, v. 50, p. 781–786.
- ↑ Potter, P. E., J. B. Maynard, and W. A. Pryor, 1980, Sedimentology of shale: Study guide and reference source: New York, Springer-Verlag, 303 p.
- ↑ Potter, P. E., J. B. Maynard, and P. J. Depetris, 2005, Mud and mudstones: Introduction and overview: New York, Springer, 297 p.
- ↑ Spears, D. A., 1980, Towards a classification of shales: Journal of the Geological Society of London, v. 137, p. 125–129.
- ↑ Stow, D. A., 1981, Fine-grained sediments: Terminology: Quarterly Journal of Engineering Geology and Hydrology, v. 14, p. 243–244.
- ↑ Macquaker, J. H. S., and A. E. Adams, 2003, Maximizing information from fine-grained sedimentary rocks: An inclusive nomenclature for mudstones: Journal of Sedimentary Research, v. 73, p. 735–744.
- ↑ 13.0 13.1 Lazar, O. R., K. M. Bohacs, J. H. S. Macquaker, and J. Schieber, 2010, Fine-grained rocks in outcrops: Classification and description guidelines, in J. Schieber, O. R. Lazar, and K. M. Bohacs, eds., Sedimentology and stratigraphy of shales: Expressions and correlation of depositional sequences in the Devonian of Tennessee, Kentucky, and Indiana: AAPG Field Guide for SEPM Field Trip 10, p. 3–14.
- ↑ 14.0 14.1 Lazar, O. R., K. M. Bohacs, J. H. S. Macquaker, J. Schieber, and T. M. Demko, 2015a, Capturing key attributes of fine-grained sedimentary rocks in outcrops, cores, and thin sections: Nomenclature and description guidelines: Journal of Sedimentary Research, v. 85, p. 230–246.
- ↑ 15.0 15.1 Lazar, O. R., K. M. Bohacs, J. Schieber, J. H. S. Macquaker, and T. M. Demko, 2015b, Mudstone primer: Lithofacies variations, diagnostic criteria, and sedimentologic/stratigraphic implications at lamina to bedset scales: SEPM Concepts in Sedimentology and Paleontology 12, 200 p.
- ↑ 16.0 16.1 Bohacs, K. M., O. R. Lazar, R. Klimentidis, J. D. Ottmann, J. Markello, M. Hardy, J. O’Leary, and J. Celerier, 2014a, Changes in mudstone properties and “shale” reservoir potential through geological time: The impact of biological and oceanic evolution.: AAPG Search and Discovery Article #90189.
- ↑ Cluff, R. M., 1980, Paleoenvironment of the New Albany Shale Group (Devonian–Mississippian) of Illinois: Journal of Sedimentary Petrology, v. 50, p. 767–780.
- ↑ Schieber, J., 1989, Facies and origin of shales from the mid-Proterozoic Newland Formation, Belt Basin, Montana, USA: Sedimentology, v. 36, p. 203–219.
- ↑ Schieber, J., 1990, Significance of styles of epicontinental shale sedimentation in the Belt Basin, mid-Proterozoic of Montana, USA: Sedimentary Geology, v. 69, p. 297–312.
- ↑ 20.0 20.1 Bohacs, K. M., 1990, Sequence stratigraphy of the Monterey Formation, Santa Barbara County: Integration of physical, chemical, and biofacies data from outcrop and subsurface, in M. M. Keller, and M. K. McGowen, eds., Miocene and Oligocene petroleum reservoirs of the Santa Maria and Santa Barbara—Venture Basins, California: SEPM Core Workshop 14, p. 139–201.
- ↑ 21.0 21.1 Bohacs, K. M., 1998, Contrasting expressions of depositional sequences in mudrocks from marine to nonmarine environs, in J. Schieber, W. Zimmerle, and P. Sethi, eds., Shales and mudstones I: Stuttgart, E. Schweizerbart’sche Verlagsbuchhandlung (Nagele u. Obermiller), p. 33–78.
- ↑ O’Brien, N. R., and R. M. Slatt, 1990, Argillaceous rock atlas: New York, Springer-Verlag, 141 p.
- ↑ Schieber, J., 1994, Evidence for episodic high energy events and shallow water deposition in the Chattanooga Shale, Devonian, central Tennessee, USA: Sedimentary Geology, v. 93, p. 193–208
- ↑ Schieber, J., 1998b, Sedimentary features indicating erosion, condensation, and hiatuses in the Chattanooga Shale of central Tennessee: Relevance for sedimentary and stratigraphic evolution, in J. Schieber, W. Zimmerle, and P. Sethi, eds., Shales and mudstones, Volume I: Stuttgart, Schweizerbart’sche Verlagsbuchhandlung (Nagele u. Obermiller), p. 187–215.
- ↑ Schieber, J., 1999, Distribution and deposition of mudstone facies in the Upper Devonian Sonyea Group of New York: Journal of Sedimentary Research, v. 69, p. 909–925.
- ↑ Macquaker, J. H. S., R. L. Gawthorpe, K. G. Taylor, and M. J. Oates, 1998, Heterogeneity, stacking patterns and sequence stratigraphic interpretation in distal mudstone successions: Examples from the Kimmeridge Clay Formation, UK, in J. Schieber, W. Zimmerle, and P. Sethi, eds., Shales and mudstones, Volume I: Stuttgart, E. Schweizerbart’sche Verlagsbuchhandlung (Nagele u. Obermiller), p. 163–186.
- ↑ Macquaker, J. H. S., S. J. Bentley, and K. M. Bohacs, 2010a, Wave-enhanced sediment-gravity flows and mud dispersal across continental shelves: Reappraising sediment transport processes operating in ancient mudstone successions: Geology, v. 38, p. 947–950. doi:10.1130/G31093.1.
- ↑ Guthrie, J. M., and K. M. Bohacs, 2009, Spatial variability of organic-matter-rich rocks: A critical element for defining the petroleum system of Pennsylvanian carbonate reservoirs of the Paradox Basin, SE Utah, in W. S. Houston, L. L. Wray, and P. G. Moreland, eds., The Paradox basin revisited—New developments in petroleum systems and basin analysis: RMAG 2009 Special Publication—The Paradox Basin, p. 95–130.
- ↑ Bohacs, K. M., J. D. Ottmann, O. R. Lazar, M. Dumitrescu, R. Klimentidis, J. Schieber, R. Montelli, C. Liu, and J. Shamrock, 2011, Genetic controls on the occurrence, distribution, and character of reservoir-prone strata of the Eagle Ford Group and related rock: Houston Geological Society Applied Geoscience Mudrocks Conference, 37 p.
- ↑ Schieber, J., J. B. Southard, and K. G. Thaisen, 2007, Accretion of mudstone beds from migrating floccule ripples: Science, v. 318, p. 1760–1763.
- ↑ Macquaker, J. H. S., M. A. Keller, and S. J. Davies, 2010b, Algal blooms and “marine snow”: Mechanisms that enhance preservation of organic carbon in ancient fine-grained sediments: Journal of Sedimentary Research, v. 80, p. 934–942.
- ↑ Krone, R. B., 1962, Flume studies of the transport of sediment in estuarial shoaling process: Final Report, Hydrological Engineering and Sanitary Engineering Research Laboratory, University of California, Berkeley, 110 p.
- ↑ Krone, R. B., 1978, Aggregation of suspended particles in estuaries, in B. Kjerfve, ed., Estuarine transport processes: Columbia, South Carolina, University of South Carolina Press, p. 177–190.
- ↑ Partheniades, E., 1965, Erosion and deposition of cohesive soils: Journal of the Hydraulics Division, v. 91, 105–139.
- ↑ Schieber, J., O. R. Lazar, and K. M. Bohacs, 2010a, Sedimentology and stratigraphy of shales: Expressions and correlation of depositional sequences in the Devonian of Tennessee, Kentucky, and Indiana: AAPG Field Guide for SEPM Field Trip 10, 172 p.
- ↑ Schieber, J., 2012, Flume experiments with carbonate muds and their relevance for the micrite problem and the deposition of ancient carbonates: AAPG Search and Discovery article #90142.
- ↑ Schieber, J., O. R. Lazar, K. M. Bohacs, B. Klimentidis, J. Ottmann, and M. Dumitrescu, 2013, An SEM study of porosity in the Eagle Ford Shale of Texas, in W. K. Camp, E. Diaz, and B. Wawak, eds., Electron microscopy of shale hydrocarbon reservoirs: AAPG Memoir 102, p. 42–66.
- ↑ Berner, R. A., 1980, Early diagenesis: A theoretical approach: Princeton, New Jersey, Princeton University Press, 241 p.
- ↑ Berner, R. A., 1981, A new geochemical classification of sedimentary environments: Journal of Sedimentary Petrology, v. 51, p. 359–365.
- ↑ Macquaker, J. H. S., and K. G. Taylor, 1996, A sequence-stratigraphic interpretation of a mudstone-dominated succession: The Lower Jurassic Cleveland Ironstone Formation, UK: Journal of the Geological Society of London, v. 153, p. 759–770.
- ↑ Macquaker, J. H. S., and C. R. Jones, 2002, A sequence-stratigraphic study of mudstone heterogeneity: A combined petrographic wireline log investigation of Upper Jurassic mudstones from the North Sea (UK), in M. Lovell and N. Parkinson, eds., Geological applications of well logs: AAPG Methods in Exploration 13, p. 123–141.
- ↑ Bohacs, K. M., and O. R. Lazar, 2010a, Sequence stratigraphy in fine-grained rocks at the field to flow-unit scale: Insights for correlation, mapping, and genetic controls: Applied Geoscience Conference of US Gulf Region, Mudstones as Unconventional Shale Gas/Oil Reservoirs, Houston Geological Society Annual Meeting, Houston, Texas, February 8–9, 2010.
- ↑ Bohacs, K. M., and O. R. Lazar, 2010b, Sequence stratigraphy in fine-grained rocks, in J. Schieber, O. R. Lazar, and K. M. Bohacs, eds., Sedimentology and stratigraphy of shales: Expression and correlation of depositional sequences in the Devonian of Tennessee, Kentucky, and Indiana: SEPM Field Guidebook, p. 15–30.
- ↑ Niklas, K. J., B. H. Tiffney, and A. H. Knoll, 1983, Patterns in vascular land plant diversification: Nature, v. 303, p. 614–616. doi:10.1038/303614a0.
- ↑ Stanley, S. M., and L. A. Hardie, 1998, Secular oscillations in the carbonate mineralogy of reef-building and sediment-producing organisms driven by tectonically forced shifts in seawater chemistry: Palaeogeography, Palaeoclimatology, Palaeoecology v. 144, p. 3–19.
- ↑ Markello, J. R., R. B. Koepnick, L. E. Waite, and J. F. Collins, 2008, The carbonate analogs through time (CATT) hypothesis and the global atlas of carbonate fields—A systematic and predictive look at Phanerozoic carbonate systems: SEPM Special Publication 89, p. 1–31.
- ↑ Carpenter, M., and C. M. Keane, 2016, The Geoscience Handbook, AGI Data Sheets, 5th ed.: Alexandria, Virginia, American Geosciences Institute, 478 p.