Difference between revisions of "Vaca Muerta play"
Cwhitehurst (talk | contribs) |
Cwhitehurst (talk | contribs) |
||
Line 117: | Line 117: | ||
===Stratigraphic Setting=== | ===Stratigraphic Setting=== | ||
− | The Vaca Muerta Formation corresponds to the distal facies of a clinoform system developed during a shallowing-upward sedimentary cycle, lasting approximately 11 My, known as the Vaca Muerta–Quintuco system<ref>Mitchum, R. M., and M. A. Uliana, 1982, Estratigrafía sísmica de las formaciones Loma Montosa, Quintuco y Vaca Muerta, Jurásico Superior y Cretácico Inferior de la Cuenca Neuquina, República Argentina: 1° Congreso Nacional de Hidrocarburos, Petróleo y Gas: Buenos Aires, Argentina, Actas, p. 439–484.</ref><ref> Spalletti, L., and G. Veiga, 2007, Variability of continental depositional systems during lowstand sedimentation: An example from the Kimmeridgian of the Neuquén Basin, Argentina: Latin American Journal of Sedimentology and Basin Analysis, v. 14, p. 85–104.</ref>. The Vaca Muerta is associated with the bottomsets and foresets of the clinoforms, consisting of organic-rich mudstones and marlstones. The Quintuco Formation represents the topsets, and consist of grainstones, wackestones, and dolostones. The progradation of the clinoforms created a thickening of the Vaca Muerta toward the northwest. It is in this vertical stack of organic-rich units where industry is landing the unconventional horizontal wells (e.g., Vittore et al.<ref name=Vttor2020 />; [[:file:M125-VacaMuerta-Figure5.jpeg|Figure 5]]). | + | The Vaca Muerta Formation corresponds to the distal facies of a clinoform system developed during a shallowing-upward sedimentary cycle, lasting approximately 11 My, known as the Vaca Muerta–Quintuco system<ref>Mitchum, R. M., and M. A. Uliana, 1982, Estratigrafía sísmica de las formaciones Loma Montosa, Quintuco y Vaca Muerta, Jurásico Superior y Cretácico Inferior de la Cuenca Neuquina, República Argentina: 1° Congreso Nacional de Hidrocarburos, Petróleo y Gas: Buenos Aires, Argentina, Actas, p. 439–484.</ref><ref> Spalletti, L., and G. Veiga, 2007, Variability of continental depositional systems during lowstand sedimentation: An example from the Kimmeridgian of the Neuquén Basin, Argentina: Latin American Journal of Sedimentology and Basin Analysis, v. 14, p. 85–104.</ref>. The Vaca Muerta is associated with the bottomsets and foresets of the clinoforms, consisting of organic-rich [[mudstones]] and marlstones. The Quintuco Formation represents the topsets, and consist of grainstones, wackestones, and dolostones. The progradation of the clinoforms created a thickening of the Vaca Muerta toward the northwest. It is in this vertical stack of organic-rich units where industry is landing the unconventional horizontal wells (e.g., Vittore et al.<ref name=Vttor2020 />; [[:file:M125-VacaMuerta-Figure5.jpeg|Figure 5]]). |
The prograding clinoforms are here subdivided into six units<ref name=Mnsni2020a /> ([[:file:M125-VacaMuerta-Figure5.jpeg|Figure 5]]). Each unit represents a transgressive-regressive sedimentary cycle of third order. The sedimentary cycles show that the highest total organic carbon (TOC) is recorded during the late transgressive systems tract (TST) and early highstand systems tract (HST), when organic matter accumulated both in the bottomset and along the foresets, forming condensed sections. During the falling stage systems tract (FSST), sediment bypassed the topsets increasing the sediment accumulation rate in the foresets, which resulted thicker and diluted in TOC. During the lowstand systems tract (LST), organic-rich intervals accumulated only in the distal bottomsets ([[:file:M125-VacaMuerta-Figure5.jpeg|Figure 5]]). The maximum thickness of the organic-rich intervals results parallel to (and basinward of) the shelf break, and the maximum area covered by the organic-rich intervals is associated with the least steep foresets<ref name=Dmngz2020 />. These observations suggest that the organic matter distribution is controlled by the construction of the clinoform and may be predicted through the sequence stratigraphy ([[:file:M125-VacaMuerta-Figure9.jpeg|Figure 9]]). Because thick units with high TOC (and associated high porosity) are fundamental elements for a landing zone, the proximal bottomsets and the lower foresets were soon indicated as zones with high hydrocarbon prospectivity ([[:file:M125-VacaMuerta-Figure5.jpeg|Figure 5]]). | The prograding clinoforms are here subdivided into six units<ref name=Mnsni2020a /> ([[:file:M125-VacaMuerta-Figure5.jpeg|Figure 5]]). Each unit represents a transgressive-regressive sedimentary cycle of third order. The sedimentary cycles show that the highest total organic carbon (TOC) is recorded during the late transgressive systems tract (TST) and early highstand systems tract (HST), when organic matter accumulated both in the bottomset and along the foresets, forming condensed sections. During the falling stage systems tract (FSST), sediment bypassed the topsets increasing the sediment accumulation rate in the foresets, which resulted thicker and diluted in TOC. During the lowstand systems tract (LST), organic-rich intervals accumulated only in the distal bottomsets ([[:file:M125-VacaMuerta-Figure5.jpeg|Figure 5]]). The maximum thickness of the organic-rich intervals results parallel to (and basinward of) the shelf break, and the maximum area covered by the organic-rich intervals is associated with the least steep foresets<ref name=Dmngz2020 />. These observations suggest that the organic matter distribution is controlled by the construction of the clinoform and may be predicted through the sequence stratigraphy ([[:file:M125-VacaMuerta-Figure9.jpeg|Figure 9]]). Because thick units with high TOC (and associated high porosity) are fundamental elements for a landing zone, the proximal bottomsets and the lower foresets were soon indicated as zones with high hydrocarbon prospectivity ([[:file:M125-VacaMuerta-Figure5.jpeg|Figure 5]]). |
Latest revision as of 20:07, 15 January 2024
Giant Fields of the Decade: 2010-2020 | |
![]() | |
Series | Memoir |
---|---|
Chapter | Geology and Economy to De-Risk the Unconventional Vaca Muerta Play, Argentina |
Author | Daniel Minisini and Fernando Sanchez Ferrer |
Link | Web page |
With willingness to invest and good geology, the Unconventional Revolution will not remain a North American story. The first areas to reach economically successful developments of unconventional resources will probably be the petroleum-rich provinces with important legacy data, substantial existing infrastructure, and public acceptance. The Neuquén Basin (Argentina) is the first place where the Unconventional Revolution has been exported with success, and the Vaca Muerta play represents the first economical unconventional play outside North America. The history of the discovery and development of the Vaca Muerta play dances for more than a decade with an intense series of events, such as the international search of new unconventional resources, the nationalization of the main operator, dramatic shifts in fiscal regime, a quick evolution of the play concepts (from vertical to horizontal wells) and of technology (from 500 to 3000 m laterals), and the Covid-19 pandemic. Nevertheless, the situation allowed conditions for economic success.
The geological knowledge of the Vaca Muerta play derives from (1) data gathered during the last decade, related to the search and discovery of the Giant Field Vaca Muerta, and (2) the legacy of 100 years of activity of the O&G industry in the Neuquén Super Basin. The integration of the geological disciplines, at different scales, presents a unique unconventional play, exceptionally thick (100–400 m), vast (30,000 km2), and porous (10%–20%), with a prograding clinoform hosting up to eight landing zones, and all types of fluid segments (from black oil to dry gas).
The current play concept consists in landing the horizontal wells in the proximal bottomsets and lower foresets of the clinoform, sectors with higher hydrocarbon potential and easier fracture growth. The rocks in these sectors show the best reservoir characteristics (averages: TOC 5%; porosity 12%; clay 10%–20%; water saturation 20%), the most adequate geomechanical properties (homogeneous rock with Young’s Modulus <4 Mpsi, low Poisson Ratio ~0.25, and interfaces with weak geomechanical contrasts), and a thick vertical stack (30–40 m) of lithofacies with the aforementioned characteristics. With this concept in mind, at least two landing zones have been fully de-risked, and other six have been confirmed.
The Vaca Muerta play has continued to progress lowering the breakeven to US$40/bbl for oil and US$2.0 MMBtu for wet gas. As activity ramps up, these values will continue to fall. The operators in the development phase believe that the combination of costs, well performance, and product prices are earning a return in their investments. In other words, the combination of below ground factors (geology) and above ground factors (contract terms, regulations, supply chain, security, market access, etc.) are allowing the development to be profitable.
Introduction[edit]
The Neuquén Basin is considered a Super Basin[1]. In fact, the Neuquén Basin is a petroleum-rich province with (1) a 7-km-thick hydrocarbon-bearing stratigraphic column with both conventional and unconventional targets (Figure 1); (2) four source rocks (Los Molles, Vaca Muerta, Pilmatué, and Agua de la Mula formations; Figure 1); (3) 9.2 Bboe (billion barrels of oil equivalent) produced (from first oil in 1918, until 2019), approximately 500 kboe/d as current production, and estimated 14.5 Bboe of proved plus probable reserves (2P) (Figure 2); and (4) substantial existing infrastructure that evacuates oil and gas to the markets of Bahia Blanca, Buenos Aires, and Chile (Figure 2).
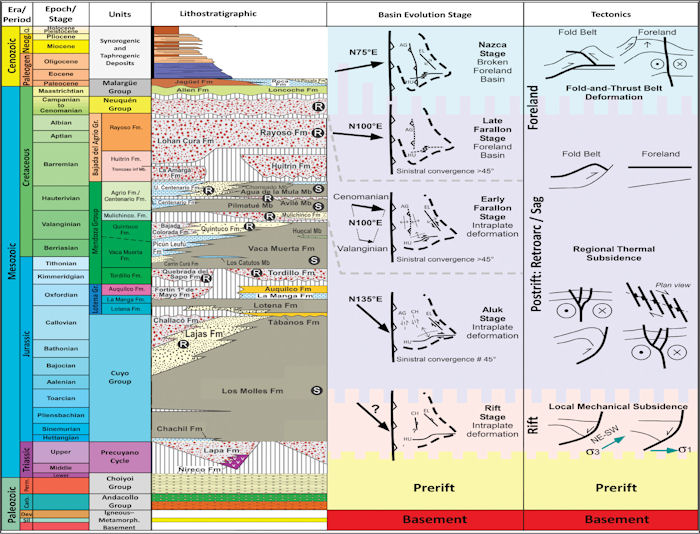
Nevertheless, the unconventional exploration and production in the Neuquén Basin is in its infancy, and the main unconventional target is the Vaca Muerta Formation (Late Jurassic). The Vaca Muerta Formation is the first economical unconventional self-sourced play outside North America; it produces from multiple fluid windows (Figure 3) and requires horizontal drilling and hydraulic-fracture stimulation to achieve per-well recoverable volumes and rates that can be developed commercially. The recovery mechanism is pressure depletion of the stimulated rock volume, and the daily production has reached 110 kbbl/d of oil and 1.1 Bcf/d of gas (32 MMm3/d; Figure 4).
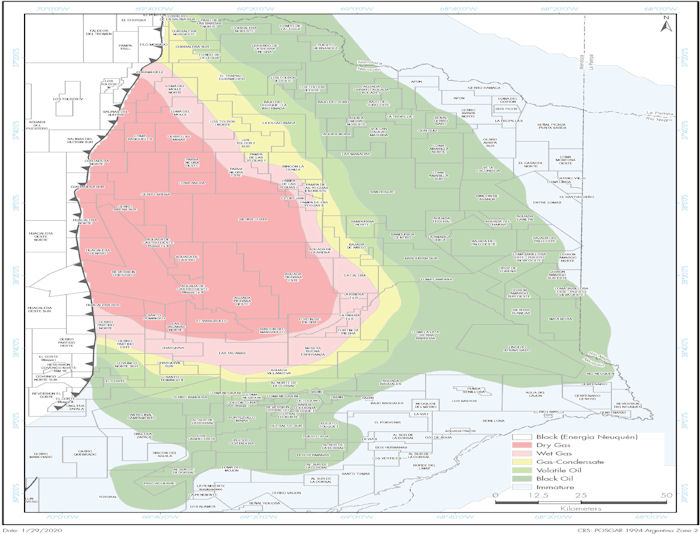
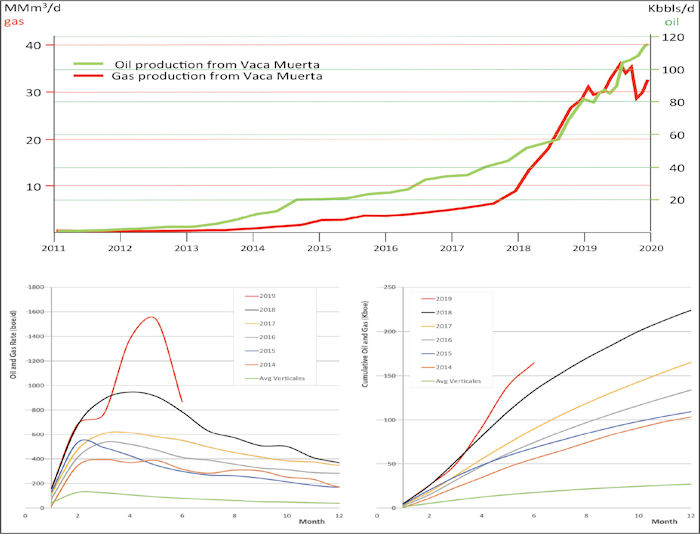
The most important decision in the exploration phase of an unconventional play is the selection of the landing zone for the horizontal well (Figure 5). The choice is based on structural studies to determine depth and avoid faulted areas, seismic stratigraphy to map the extension of the targeted unit, sedimentology and sequence stratigraphy to predict the lateral facies variability, geochemistry to determine the fluid segment, and petrophysics and geomechanics to define the rock properties. The selection of the landing zone, and the associated understanding of the resource distribution, is important because it anchors the development phase with repeatability and high confidence. As a consequence, during the phases of exploration, pilot, and appraisal, it is not vital to find multiple landing zones, one is enough. The analyses to determine lateral length of the horizontal well, fracture intensity of the hydraulic fracturing, and distance between wells in a pad design are focused on the completion optimization to enhance production. In fact, it is commonly believed that reservoir quality accounts for 70%–80% of unconventionals’ successes, and completion accounts for 20%–30%.

History of the Discovery and Development of the Vaca Muerta Play[edit]
In the first years of the 21st century, the development of the unconventional plays in North America (i.e., North Dakota, Pennsylvania, Texas) encouraged the O&G industry to screen proved source rocks around the world to expand the frontiers of the new unconventional resources. Considering geochemical parameters, pay thickness, drilling depths, acreage, and availability of infrastructure, the Vaca Muerta Formation emerged as an ideal candidate to start unconventional exploration outside North America. In fact, legacy wells proved flows of natural gas and oil at economic rates in units affected by natural fractures, geologic maps documented a large area of potential resources (30,000 km2 [11,583 mi2]), and data suggested three distinct, highly prospective thermal maturity windows (oil, condensate, and gas). Furthermore, the Neuquén Basin presented extra elements favorable for the development of an unconventional play: flat geomorphology, low population density, easy water access, limited competition for surface use and existing oil and gas infrastructure.
Major conventional oil and gas fields were discovered in Neuquén between the 1960s and the 1980s, among them, the giant field Loma La Lata (12 Tcf) and El Trapial (500 mmbl). Production in the basin, with historic values of 300 kbbl/d of oil and 2.8 Bcf/d of gas, reached a peak in the early 2000s. However, by 2009–2010, the conventional sector in Neuquén was in rapid decline. In 2007, the licensing for exploration was transferred from the federal to the provincial governments, and the Province of Neuquén encouraged the development of the Vaca Muerta play. The first leasing acreage occurred in December 2009, when three blocks in the core of the play (Bajada de Añelo, La Escalonada, and Rincón de la Ceniza) were awarded in an open bid round (Figure 3). In October 2010, a new bid round for 13 remaining blocks in the prospective area were awarded, mostly to companies that already had a presence in the basin. The subsequent acreage acquisitions occurred mainly through joint venture agreements.
In late 2010, Repsol-YPF, the main operator in the basin, drilled and hydraulically fractured the first vertical wells aimed at evaluating the potential of Vaca Muerta as a self-sourced unconventional play, announcing the discovery of 927 MMboe of recoverable resources. The resource consisted of good quality shale oil, with an API of 40°–45°, covering an area of 428 km2 in the Loma la Lata–Sierra Barrosa block, where legacy data indicated optimal conditions to start the venture (several wells with gas kicks and hydrocarbon flows). The vertical wells tested a 250-m (820-ft)-thick target, completed with four to five fracture stages per well, and produced at encouraging initial rates of 200–500 barrels/day, which matched recoveries attained by some horizontal producers in North America. These results, together with a report sponsored by the U.S. Energy Information Administration[8], listing the Vaca Muerta as the most prospective gas shale in the world (with 308 Tcf of recoverable natural gas) and the second most prospective oil shale (with 16.2 Bboe of recoverable oil), attracted the international companies, and the exploration of the Vaca Muerta play took off in 2011, with the potential to totally reverse the declining production trend observed in Argentina since 2002.
The initial development concept was that the extraordinary pay thickness (100–400 m) was enough for vertical wells with multiple fracture stages, but very soon the concept was revised, and the first horizontal wells were drilled, significantly increasing production. In 2012, the first multiwell pad (generally three or four wells per pad) dramatically scaled up the drilling technology, minimizing the environmental footprint and reducing the number of rigs needed. Since 2014, drilling activities started shifting from vertical to horizontal wells, with the latter now dominating basin production and new projects. At the beginning of 2020, 1225 wells had been drilled in the Vaca Muerta play, approximately 900 are in production, half of which are horizontal.
In 2012, after the expropriation and re-nationalization of YPF (then owned by the Spanish oil major Repsol), the government of Argentina launched a growth program driven by the Unconventional plays in the Neuquén Basin (tights sandstones and shales). Investment had fallen across the sector since 2001 because of a government policy of freezing domestic oil and gas prices and limiting exports, pushing Argentina to become a net importer of both oil and natural gas by 2011. That year, the gap reached 425 mmscf/d, which had to be filled with costly imports from Bolivia and Liquefied Natural Gas (US$11–17/MMBtu), whereas local producers received about US$3/MMBtu. In response to the crippling gas-import bills, the Gas Plus plan granted an elevated gas price of US$7.5/MMBtu for incremental new gas, significantly improving netbacks for companies with new shale gas and tight gas discoveries. In 2014, the Federal Hydrocarbon Law was amended to allow an Unconventional Exploitation Concession of 35 years, five of which dedicated to determining the commerciality of the block. This new regulation provided a robust framework for long-term investments and a considerable flexibility in the early years. Therefore, since 2014, fueled by industry investment, the production from the Vaca Muerta play has dramatically ramped-up, reaching approximately 110 kbbl/d of oil and 1.1 Bcf/d of gas (32 MMm3/d) by the beginning of 2020 (Figure 4), providing 20% and 23% of the national oil and gas supply, respectively. This supply has virtually eliminated the need for oil imports in the country (but not for gas, because of the strongly seasonal character of the demand). The volumes of hydrocarbon produced and the estimated 14.5 Bboe of proved plus probable reserves (2P) make of the Vaca Muerta unconventional play a Giant Field, although it should be better defined as Giant Accumulation because of its unconventional nature[9].
The governmental New Plan Gas presented in 2017 guaranteed a special price for gas only for the companies producing above a certain amount. The special price gradually decreases from 7.5 US$/MMBtu in 2018 to 6 US$/MMBtu in 2021. At the beginning of 2020, the production from the Vaca Muerta unconventional play consists of 40% oil and 60% gas (on energy equivalent basis). However, at present (2021), production is shifting from gas to oil because of the low wellhead gas prices and the oversupply of natural gas (Figure 4).
Currently, the Vaca Muerta play has 25 projects in pilot phase and 6 in development phase: that is, Loma Campana (black oil) by YPF-Chevron; El Orejano (dry gas) by YPF-Dow; Fortin de Piedra (dry gas) by Tecpetrol; Aguada Pichana Este (dry gas) by Total and others; La Amarga Chica (black oil) by YPF-Petronas; and Sierras Blancas, Cruz de Lorena, CASO (black oil) by Shell. Bajo del Choique (volatile oil) by Exxon and La Calera (gas condensate) by Pluspetrol may soon move to the development phase (see blocks in Figure 3). However, note that this contribution was printed during the Covid-19 pandemic, the biggest health and economic crisis in decades, a moment of highest uncertainty for the future of society, industry and the environment.
Geology, Technology, and Experience[edit]
In general, Argentina’s unconventional development has paralleled its evolution in the United States, but at a smaller scale. Considering investment, for example, American plays such as the Eagle Ford and Bakken were receiving US$20–40 billion per year for drilling and completion, whereas about US$10 billion have been spent in Vaca Muerta from 2011 to date. Regarding learning, most plays in the United States relied on a model of knowledge-sharing among multiple operators working simultaneously (sometimes up to 50) and providing regular publicly available updates to investors, thereby increasing information flow and accelerating learnings for the O&G industry as a whole. Instead, the early days of the Vaca Muerta were heavily dominated by YPF, which was thus compelled to increase its associations with partners, signing up with Chevron in 2012, Dow in 2013, Petronas in 2014, and Shell in 2017.
To unravel the unknown geological aspects of the Vaca Muerta Formation, geologists took advantage of the new data sets collected, integrating several disciplines at different scales (i.e., structural geology, stratigraphy, sedimentology, petrography, geochemistry, petrophysics, geomechanics; e.g., González et al.[10]), hence defining, mapping, and ranking the key areas and stratigraphic zones where to land the horizontal wells. The most prospective areas were identified in the Embayment sector (the entire area shown in Figure 3), and the most prospective landing zones were identified, first, in the lowermost units of the Vaca Muerta Formation of the southern sector (Unit 1 and Unit 2) and, later, in the upper units of the northern sector (Unit 3 and Unit 4; Figure 5). This allowed operators to increase productivity and repeatability within the first few years of exploration.
By early 2012, YPF had brought into production 20 vertical wells and 4 horizontal wells in the Loma Campana block, with most production consisting of black oil from geomechanical-competent intervals (thus considered more frackable) in the upper Unit 1 (aka Regresivo). The potential of the muddier lower Unit 1 (aka Cocina) was revealed by Shell in early 2013, in the Sierras Blancas block, with a 500-m horizontal well having good initial production (~600 bbl/d), hence dispelling the notion that the lowermost Vaca Muerta was too ductile for efficient hydraulic fracturing. In mid 2014, a 1500-m horizontal well from Exxon, in Bajo del Choique block, proved impressive oil production rates (~770 bbl/d) in Unit 4, hence reinforcing the idea of potential multiple landing zones (Figure 5).
Simultaneous to the geological breakthroughs, there was a continuous technological progress. Switching from verticals to horizontals wells allowed operators to expand the number of frac stages (from 3 up to 54). Completions were performed more intensively (currently ~3000 pounds of proppant per foot) and lateral lengths increased over the years (from 500–1500 m before 2014, to the current 2000–2500 m, with a handful of wells in the basin attaining 3000–3300 m). These technological improvements translated in an increase of the estimated ultimate recovery (EUR) of 15%–16% per year, for the last 3–4 years. Currently, EUR’s are estimated 0.5–1.3 MMboe/well in the black oil window and 11–16 Bcf/well in the dry gas window (assuming 2500 m laterals).
The technological progress made the wells more productive, whereas the gained experience in the development phase made the wells cheaper. In fact, drilling and completion well costs fell as rapidly as the North American unconventional plays, averaging 10%–12% per year: from US$20–25 million/well with a 500 m lateral in 2012, to US$11–12 million/well with a 2500 m lateral in 2020. Materially contributing to these improvements was the switch in proppant, from ceramics to sand, and then the reduction in the cost of sand, which has dropped approximately 30% since 2015, when it started to be mined in Argentina, instead of being imported from Brazil, China, or the United States.
In summary, geological knowledge, technology, and experience allowed operational costs (Opex) to drop 15%–20% per year (2017–2019), to the current US$8/boe production. Although these significant gains will tend to taper off, the total cost to produce in Vaca Muerta (Capex + Opex) may decrease to US$16/boe within the next 1–3 years.
Local, Regional, and Global Economic Factors[edit]
The development breakeven prices (BEP) for the Vaca Muerta play have benefited from the aforementioned trends of lower cost and higher productivity. Breakeven prices for oil are currently (2020) US$40–45/bbl, down from US$60 only four years ago, which makes Vaca Muerta nearly as competitive as North American unconventional plays (BEP: 30–35$/bbl). Breakeven prices for dry gas are US$3.5–4.0 $/MMBtu, which makes Vaca Muerta dry gas less competitive than North American unconventional plays (BEP: US$2.3/MMBtu). However, Vaca Muerta wet gas economics benefit considerably from the presence of condensates (C8+), which in Argentina are valued like at oil prices (minus a few-dollar discount). Hence for wet gas, BEP could be around 1.5–2.0$/MMBtu (60$/bbl oil price assumed for this estimation).
The activity in the Vaca Muerta play is changing the gas-market dynamics in the region. In 2018, at the peak of Vaca Muerta’s gas surge, the government of Argentina announced that unconstrained gas exports to Chile could take place once the internal demand was satisfied. However, because of the strong seasonal variations in Argentinian gas demand (~1.7 Bcf/d), this option is likely to be restricted to the Austral summer when internal demand is low, and LNG imports from Chile will still be needed during the high demand in the Austral winter (May to September). Furthermore, the actual need of gas in Chile is limited to approximately 600 MMcf/d, which means that Argentinian gas producers will likely need to shut-down wells in the Austral summer until a long-term export solution is established (i.e., plants of Liquefied Natural Gas, LNG), or plans to build extra storage capacity are executed.
After gas production from Vaca Muerta quadrupled from 2017 to 2019, growth slowed considerably because of low wellhead prices. In fact, after a major financial crisis hit Argentina in 2018, the government restricted price incentives, slowed their awards, and revamped export duties (implemented by previous administrations prior to 2016). Downward pressures on baseline gas-prices because of increased supply were compounded with a significant devaluation of the Argentinian currency in 2018, leading ultimately to value erosion of domestic gas sales.
The growth of gas production slowed also because of the emergence of midstream bottlenecks. In fact, there are three gas pipelines connecting Neuquén with the largest gas market around Buenos Aires and two export pipelines to Chile, with a total pipeline capacity close to 3 Bcf/d. Considering that Neuquén gas production (conventional and unconventional) reached 2.8 Bcf/d in August 2019, it follows that ullage (unused capacity) is limited and may soon be restricted to the Austral summer. Expansions of domestic transport capacity and investment in separation and export facilities will be required to sustain Vaca Muerta gas growth in the future. Several private initiatives are currently addressing this need, including increasing the connection of Neuquén’s large gas segment to trunk transmission systems.
In response to the low wellhead prices and the midstream bottlenecks, operators are shifting their attention back to the liquids. The oil fluid-segment offers competitive BEP and baseline prices on parity with international markets. Furthermore, some margin of unused capacity for oil evacuation exist in the Oldelval pipeline (capacity of 220,000 bbl/d), which transport the oil produced in the Neuquén Basin to the port facility of Bahía Blanca (513 km apart). However, also in the oil midstream, upgrades and investment are needed to sustain future production growth.
An LNG export solution is increasingly seen as the way forward to sustain the growth of gas production in Vaca Muerta and to enhance growth in oil production, with its significant quantities of associated gas. In mid-2019, YPF became an LNG exporter, with the small-scale floating liquefaction unit Tango FLNG (0.5 MMtpa). LNG export solutions, however, are challenged by the strong seasonality of Argentinian gas demand, which will likely lead to plant underuse and higher liquefaction costs during the Austral winter, when the increased needs of the domestic market have priority over exports. At the same time, Argentinian LNG exports could benefit from “counter seasonal” demand increases in the northern hemisphere, as excess supply during the Argentinian summer months (October to April) coincides with peak demand in Asia. Another option to sustain the growth of gas production would be to build extra storage capacity for the underused gas during the hot summer months.
Vaca Muerta’s increased production could sustain LNG plants reaching 6 MMtpa by 2024 and 10 MMtpa by 2030[11]. If sufficiently rich in ethane and other Natural Gas Liquids (i.e., propane, butane), the gas production could supply an ethane cracker of 1–2 million tonnes, with its polyethylene derivatives. These monetization opportunities (LNG export and chemicals) will offer Vaca Muerta the possibility to create an integrated value chain, typical of the profitable developments of the North American unconventional plays.
Presently, in 2021, the Vaca Muerta play is at a critical juncture. Multiple companies are entering the development phase and the basin could experience high levels of investment and activity, as it happened in the North American unconventional plays. Forecasts by some consultancy groups anticipate productions of 1 MMboe/d by 2025. However, the region still needs to overcome several issues to fulfill these expectations and spark broader development, including political risk, access to capital, improvement of relations between unions and the private sector, infrastructure bottlenecks, limitations in oil services and supply chain. Moreover, a factory mode development scenario may imply doubling the number of rigs in the basin (currently around 35) as well as the availability of proper equipment and proppant. These elements would imply environmental and social challenges, including competition for land use and transport infrastructure, as well as water and air management.
Conditions for Economic Success[edit]
The Vaca Muerta play has continued to progress lowering the breakeven to US$40/bbl for oil and US$2.0 MMBtu for wet gas. As activity ramps up these values will continue to fall, placing the Vaca Muerta play squarely on its way to be a disrupter in Argentina’s O&G industry, the economy of Argentina and of the Southern Cone. The operators in the development phase believe that the combination of costs, well performance, and product prices are earning a return in their investments. In other words, the combination of below ground factors (geology) and above ground factors (e.g., contract terms, regulations, supply chain, security, market access) are allowing the development to be profitable. Although several other organic-rich formations around the world (in China, Australia, Russia, Saudi Arabia, Oman, Mexico, Indonesia) may have comparable potential to the Vaca Muerta play, the presence of several critical factors has allowed the latter to become the first competitive unconventional self-sourced play outside of North America (see Table 1).
Repsol-YPF fractured the initial Vaca Muerta vertical wells in 2010, hence it has taken the O&G industry some 10 years and 1000 wells, to prove the play’s competitiveness with North American resources. And it has taken 3–7 years for major O&G companies to become confident enough in its commerciality, to sanction large developments. With time, willingness to invest and good geology, the Unconventional Revolution will not remain a North American story and Vaca Muerta will be just the first example of economically successful unconventional development, among other petroleum-rich provinces endowed with infrastructure (known as Super Basins).
Phases to De-Risk an Unconventional Play: Exploration, Appraisal, Pilot, and Development In the Vaca Muerta play, as in similar worldwide unconventional plays, the realization of an opportunity occurs through the successive phases of exploration, appraisal, pilot, and development, where each phase brings new understanding of the play, but at the same time increasing capital exposure (Figure 6). The exploration phase aims to validate the play concept demonstrating the occurrence of hydrocarbon flow from the unconventional reservoir. During the exploration phase, the focus is on rock analyses, which derive from legacy wells, competitor information, and actual drilling of vertical wells. The resulting data produce maps that assess the play emphasizing the key subsurface parameters, in turn, indicating the best areas (sweet spots) and the best stratigraphic units (landing zones) to drill horizontal wells. Commonly, surface constraints (e.g., infrastructures, urban development, geomorphology) reduce the accessibility to those areas. The outcome of this exploration phase is a de-risking strategy (e.g., how much will likely be produced from a certain area) and a field development plan (e.g., how many wells will be drilled). The appraisal phase aims to demonstrate sustained hydrocarbon production at (near) economic rates with a clear path forward to profitable EURs. The appraisal phase drills horizontal wells into the selected landing zones understanding fracture barriers, and rock mechanics, and collects sufficient data to determine if a selected area presents (1) enough profitability to be competitive in a company’s portfolio and (2) an actionable risk-mitigation plan. The pilot phase consists of drilling an increasing number of horizontal wells with more fracture stimulations and producing over a longer time to establish production profiles, hence deriving EUR of wells with high confidence, and defining the well-to-well interaction (interference) to check the development plan. During this phase, landing zones are optimized, and facilities that process and evacuate the produced hydrocarbons are built. The development phase consists of drilling, completing, tying in, and producing an even larger number of wells, in factory mode, as well as in managing the general operation of the processing facilities over their life cycle. Infrastructure construction is also part of this phase, and, besides central production facilities, construction includes in-field gathering systems and well pad facilities. This is the phase focused on delivering cash flow.
Integrated Geology of the Vaca Muerta Formation[edit]
The following section summarizes the geological knowledge on the Vaca Muerta Formation derived from (1) the data gathered during the last decade, related to the search and discovery of the Giant Field Vaca Muerta, and (2) the data legacy of an entire century, related to the activity of the O&G industry in the Neuquén Super Basin. Both data sets are fundamental to build reliable models for planning the expensive phases needed to de-risk a self-sourced unconventional play (from the exploration phase to the development phase).
Tectonic Setting[edit]
The Neuquén Basin, located in west-central Argentina, is a sub-Andean, retroarc-related foreland depocenter, developed during the Jurassic, Cretaceous, and Cenozoic. The Mesozoic sedimentary column (7000–10,000 m thick) is characterized by four main tectono-sedimentary episodes (Figure 1): (1) the Late Triassic to Early Jurassic rift stage that recorded volcanic epiclastic deposits partly filling the dense mosaic of small half-grabens (10–70 km by 5–10 km) created during the rift itself (Precuyano Cycle); (2) the Early and Middle Jurassic thermal subsidence (Cuyo and Lotena groups) that gradually generated a single broad basin: the Neuquén Basin; (3) the Late Jurassic to Late Cretaceous thermal subsidence of the sag stage (Mendoza and Bajada del Agrio groups), with local tectonic inversions; and (4) the Late Cretaceous to Neogene retro-arc shortening (Andean uplift) that created fold-and-thrust belts, a foreland basin and a peripheral bulge (Neuquén and Malargüe groups)[12]. The tectono-sedimentary episode 3, from early Tithonian to early Valanginian, includes the deposition of the Vaca Muerta Formation over an area of 30,000 km2.
Analyzing the distribution and interaction between the inherited and the neo-formed structures, the Vaca Muerta Formation can be subdivided into four main areas[13] (Figure 7): (1) the eastern area dominated by normal faults (en echelon clusters 50–70 km long) generated by the oblique-divergent reactivation of N120°E half-graben bounding normal faults, (2) the southwestern area where en echelon normal faults are mixed with the reverse faults of the Huincul High, (3) the central western area dominated by post-depositional thrust faults rooted in the evaporitic Auquilco Formation, and strike-slip faults, (4) the northwestern area where normal fault families are affected by Cenozoic volcanic systems.
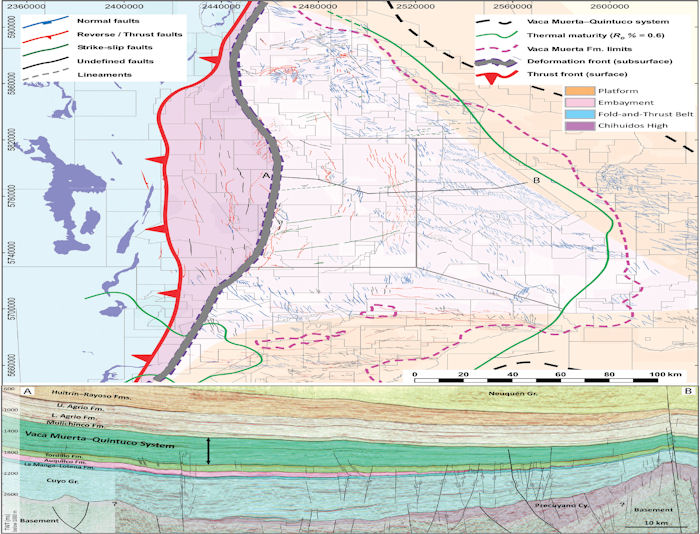
The structural characterization has an important role in the risk analysis of the Vaca Muerta play: that is, (1) most of the faults affecting the Vaca Muerta affect also Tordillo (Figure 7), hence the faults are uncontained (sensu Stephenson and Coflin[14]) and they have negative effects on production; (2) severe or total mud losses have been reported when the horizontal wells cross seismically visible faults, irrespective of the fault type or orientation; (3) canalization of the hydraulic fracturing within the planes of large-scale faults and their associated fracture networks resulted in a significant limitation of the stimulated rock volume (SRV); (4) high levels of mantellic CO2 (>5%–10%) occurred in association with deep (synrift) rooted faults and with igneous sills causing mud losses and creating strong mechanical interfaces that affect the efficiency of the hydraulic fracturing[13].
Basin Paleo-Configuration[edit]
The main structural elements during the deposition of the Vaca Muerta Formation define two types of morpho-structural domains, which roughly overlap with the four main structural areas shown in Figure 7: (1) elongated regional paleo-axes of subsidence (determining depocenters) and (2) paleo-highs (determining areas of thinner stratigraphic record): passive highs produced by relatively slower subsidence than the surrounding areas, and active highs showing progressive deformation as a result of the uplift of the Huincul High, whose activity caused steeper clinoforms, and triggered greater lateral variability in the stratigraphic architecture. This basin paleo-configuration helps understand that the location, shape, and mineralogy of the Vaca Muerta depocenters are related to the location and growth of the structural elements, which affected the sediment distribution through: (1) the northward transport of siliciclastic extrabasinal sediment from the highs of the Southern Flank, (2) the deposition of calcareous intrabasinal sediment along the ramps of the Eastern Flank, and (3) the sedimentation of volcaniclastic extrabasinal material along the Western Flank, now uplifted in the Andes (Figure 8A).
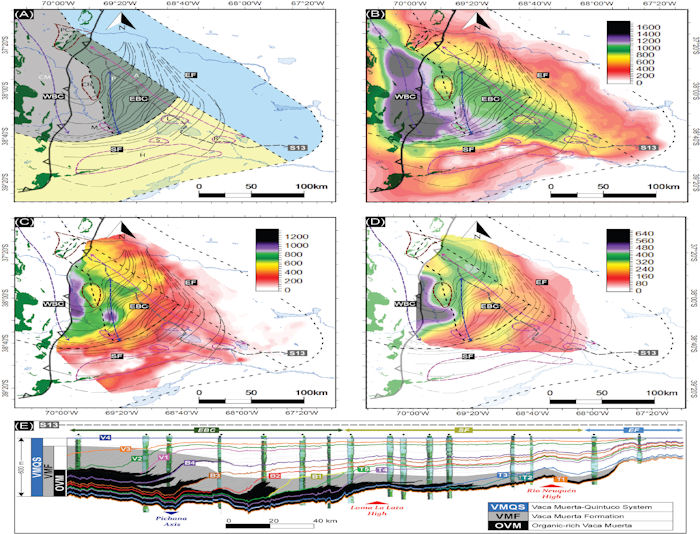
Stratigraphic Setting[edit]
The Vaca Muerta Formation corresponds to the distal facies of a clinoform system developed during a shallowing-upward sedimentary cycle, lasting approximately 11 My, known as the Vaca Muerta–Quintuco system[16][17]. The Vaca Muerta is associated with the bottomsets and foresets of the clinoforms, consisting of organic-rich mudstones and marlstones. The Quintuco Formation represents the topsets, and consist of grainstones, wackestones, and dolostones. The progradation of the clinoforms created a thickening of the Vaca Muerta toward the northwest. It is in this vertical stack of organic-rich units where industry is landing the unconventional horizontal wells (e.g., Vittore et al.[5]; Figure 5).
The prograding clinoforms are here subdivided into six units[6] (Figure 5). Each unit represents a transgressive-regressive sedimentary cycle of third order. The sedimentary cycles show that the highest total organic carbon (TOC) is recorded during the late transgressive systems tract (TST) and early highstand systems tract (HST), when organic matter accumulated both in the bottomset and along the foresets, forming condensed sections. During the falling stage systems tract (FSST), sediment bypassed the topsets increasing the sediment accumulation rate in the foresets, which resulted thicker and diluted in TOC. During the lowstand systems tract (LST), organic-rich intervals accumulated only in the distal bottomsets (Figure 5). The maximum thickness of the organic-rich intervals results parallel to (and basinward of) the shelf break, and the maximum area covered by the organic-rich intervals is associated with the least steep foresets[15]. These observations suggest that the organic matter distribution is controlled by the construction of the clinoform and may be predicted through the sequence stratigraphy (Figure 9). Because thick units with high TOC (and associated high porosity) are fundamental elements for a landing zone, the proximal bottomsets and the lower foresets were soon indicated as zones with high hydrocarbon prospectivity (Figure 5).
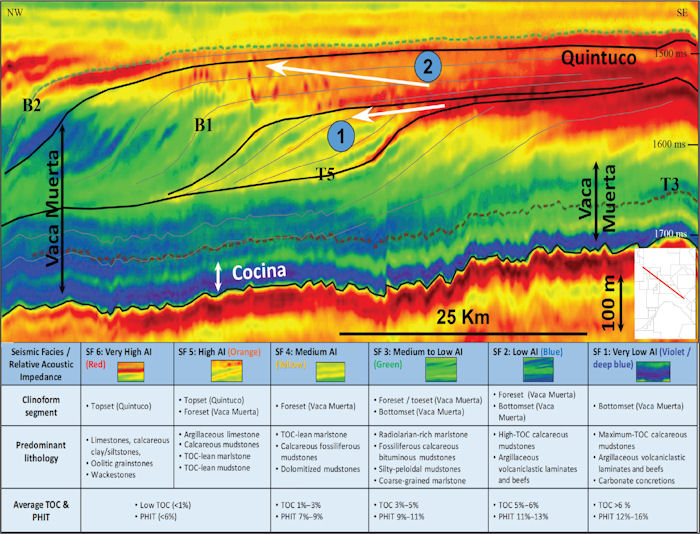
Depositional Model[edit]
The lithofacies distribution is neither homogenous nor random along the clinoform. In fact, after the integration of cores and seismic data, it becomes evident that same lithofacies re-occur within the same depositional sector (i.e., bottomset, foreset, topset) generating lithofacies motifs. The motif in the bottomsets presents an alternation of thin limestones (10–30 cm) and thick organic-rich mudstones (10–100 cm) with embedded carbonate concretions (20 × 60 cm) and numerous thin ash beds (aka tuffs or bentonites) (~1 cm). The motif in the foresets shows ripple-laminated coarse-grained marlstones and limestones with local mass-transport deposits. The motif in the topsets is characterized by grainstones and dolostones with local oolitic bars and carbonate buildups (Figures 10, (11).
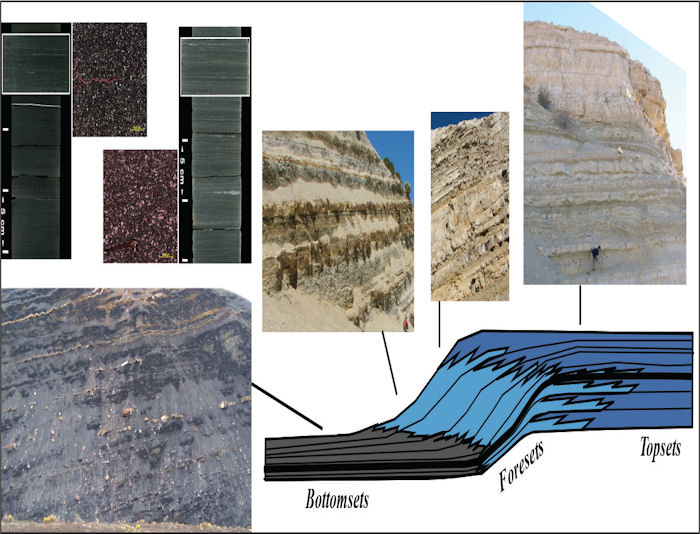
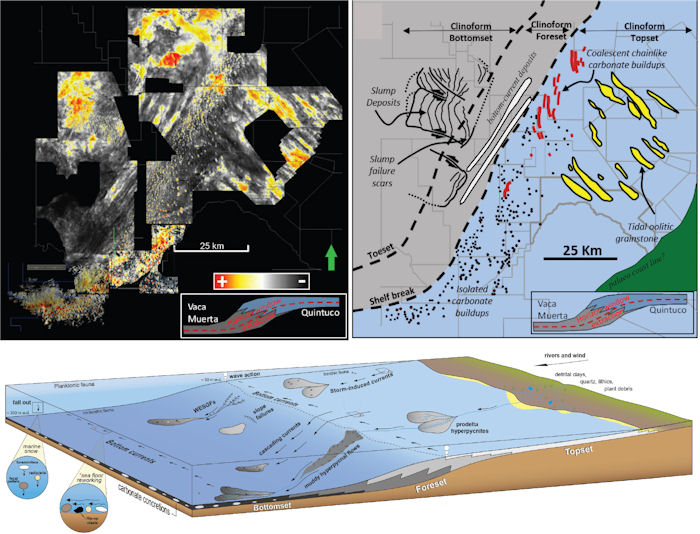
The very first deposits of the Vaca Muerta–Quintuco system overlay the continental Tordillo Formation that represented a low-relief surface when the instantaneous transgression of the ocean flooded the Neuquén Basin. During the sea level rise, the space of accommodation increased, and sediment was delivered from the margin toward the basin generating a low-angle ramp (0.2–0.3°, Units 1 and 2, representing the main landing zones). Once the sediment filled the accommodation space, the hydrodynamic processes (e.g., tides, waves, currents, gravity flows) became a key factor reworking the sediment basinward and reshaping the ramp into a sigmoidal clinoform with steep foresets (1°–3°). The increased clastic input, derived by wind and rivers, generated a mixed carbonate–siliciclastic system. When a distinguishable shelf break was created, salinity and density contrast in the water masses enhanced the current activity triggering hydrodynamic accumulations in the distal topset (banks, shoals, and in situ biohermal buildups). Banks and buildups formed incipient lithified margins suggesting that nutrient levels and currents were suitable for widespread biological growth and extensive early cementation. The preservation of organic matter in the topsets and upper foresets was poor because of the erosional currents, the high bioturbation, and the oxygenated seafloor. Instead, the lower foresets and bottomsets preserved organic-rich sediment because in depositional sectors with recurrent periods of low sediment accumulation rate, basinwide suboxia–anoxia and high bio-productivity, generally co-occurring during sea level transgressions (Figure 11). The calibration of the rock description with quantitative analyses indicates the bottomsets and lower foresets as the most suitable depositional sectors to find lithofacies with desired petrophysical characteristics (high TOC, high porosity, low water saturation, moderate clay content) and geomechanical elements (low stiffness and interfaces with weak geomechanical contrasts, hence prone to hydraulic fracturing).
Petrography[edit]
Bottomsets and lower foresets present several lithofacies generally lumped into mudstones, limestones, and ash beds. The mudstones and ash beds decrease up section and landward, vice versa the limestones. Most mudstones and limestones contain a mixture of similar components, although in different quantities. The main components are biogenic carbonate that originated in the topsets from benthic fauna and in the water column from planktonic fauna; biogenic phosphate from brachiopods, fish bones and scales; biogenic quartz from siliceous radiolarian; intrabasinal rip-up clasts created by bottom currents; micrite, probably derived from the nannofossils and fragments of skeletal grains. Detrital siliciclastic material (quartz, feldspars, micas, illite, and illite–smectite mixed layer) entered the basin by wind and rivers, whereas volcaniclastic material by air fallout. Diagenetic clays derived from the metastable volcaniclastic material, diagenetic quartz derived from the carbonate-replaced radiolaria or the progressive transformation of smectite to illite. Pyrite formed from the abiotic reactions of iron and reduced sulfur in the organic matter. Organic matter has phytoplankton and algal-bacterial origin. Cements are mainly represented by calcite, dolomite, and microgranular quartz[20].
The mudstones in the bottomsets that represent the reservoir are massive to parallel–laminated; their main components are bioclastic debris, coccolith fragments, silt-size quartz, and plagioclase grains; cements are microgranular quartz and granular calcite; mineralogy records 45% siliciclastic, 30% carbonate, 20% clay; porosity is 6%–15%, mainly in the organic matter, in leached feldspars, and within coccolith pellets; TOC ranges 4%–14%. The limestones in the bottomsets consist of planar and cross-laminated packstone/grainstone with pellets, micritic intraclasts, and calcitized radiolarian; mineralogy records 65% carbonate, 20% siliciclastic, 10% clay; porosity is 2%–4%, mainly intra-granular and intergranular; average TOC is 1%. These two lithofacies alternate in the stratigraphy of the bottomsets with a mudstone/limestone ratio of 8-to-1, and this lithofacies motif is associated with transgressions (TST and early HST), which are enriched in siliciclastic material and TOC with respect to regressions (late HST, FSST, and LST; Figure 9). Regressions show higher recurrence of limestone beds, and mudstones impoverished in TOC[18]. The transgressive-regressive cycles considered represent third-order sequences, 10s-of-meters thick in the bottomsets, and they include both landing zones (associated with transgressions) and buffer zones hampering the growth of the hydraulic fractures (associated with regressions). The understanding of this dichotomy allows an informed decision on where to land the horizontal well (generally allowing a 5–10-m-thick window to geosteer the drill bit).
Petrophysics[edit]
Porosity in the Vaca Muerta (4%–14%, up to 20%) is higher than in other self-sourced unconventional plays and it can be divided into interparticle, intraparticle, and organic-hosted[20][21]. Organic-hosted pores are the most numerous, decrease upsection (together with the decrease of the TOC), range between 10 nm and 100 μm, and are present both in kerogen and bitumen. In turn, kerogen and bitumen fill the interparticle and intraparticle pores (as well as natural fractures, if present). Interparticle pores are common, range from 10s of nanometers, between clay booklets, to 10 μm between quartz, feldspars, bioclasts, and coccolith particles. Intraparticle pores are not abundant, are poorly connected, occur within coccolith pellets, clay peloids, intraclasts, dissolved feldspars, and dissolved carbonate grains; the smallest pores (<5 nm) occur within clay booklets, the largest pores (up to 500 μm) occur within leached feldspars of detrital origin.
The origin and preservation of high porosity, that created the extraordinary storage capacity in the Vaca Muerta, result from a combination of (1) the original composition of the sediment (high TOC with organic-hosted pores, abundant pellets with intraparticle pores, organic matter fueling redox reactions and creating dissolution, feldspars dissolving and creating more storage capacity); (2) the sedimentary processes (fallout of calcareous bioclastic material and hyperpycnal flows delivering siliciclastic and volcaniclastic grains); (3) seafloor and water column chemistry (temporary pulses of anoxia and reductive conditions) triggering early diagenesis (from the metastable organic matter and volcaniclastic material); (4) reduced compaction related to early cementation. The presence of early cement and dissolution fabrics indicates that significant volumes of fluid flowed through these rocks, regardless of their low permeability, hence affecting their pore infill history.
Water saturation presents an average over the entire Vaca Muerta Fm of approximately 40%. Indication of production of formation water derive from (1) tracer’s dilution, (2) changes in isotopic signatures over time, and (3) wells with more than 100% of recoverable flowback water. Although the source of formation water is not yet understood, data document that wells in the eastern sector of the basin or landed in the Unit 1 produce less percentage of water. In average, formation water salinity is 220 ppk NaCl equivalent[22].
The Vaca Muerta Formation with dominant organic-rich lithofacies (>2% TOC) may be up to 270 m thick. Median values within this organic-rich Vaca Muerta are 11% total porosity, 44% water saturation, 5% TOC, 28% carbonate, 30% QFP, 21% clay[21]. However, the median values need to be scrutinized with accuracy because reservoir properties and fluid types vary significantly both among the six units of the Vaca Muerta-Quintuco system and within each unit (Figure 12). These variations suggest that each stratigraphic interval of interest must be addressed and analyzed as a different play type, with potentially different hydrocarbon storage, response to stimulation, and productivity (Figure 13).
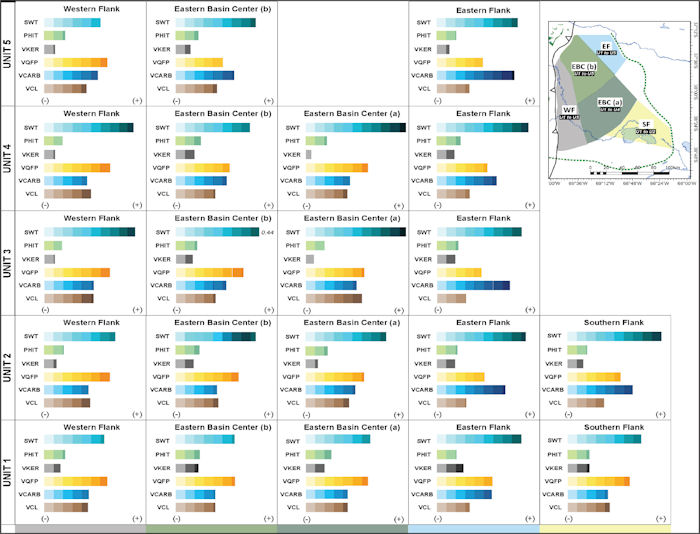
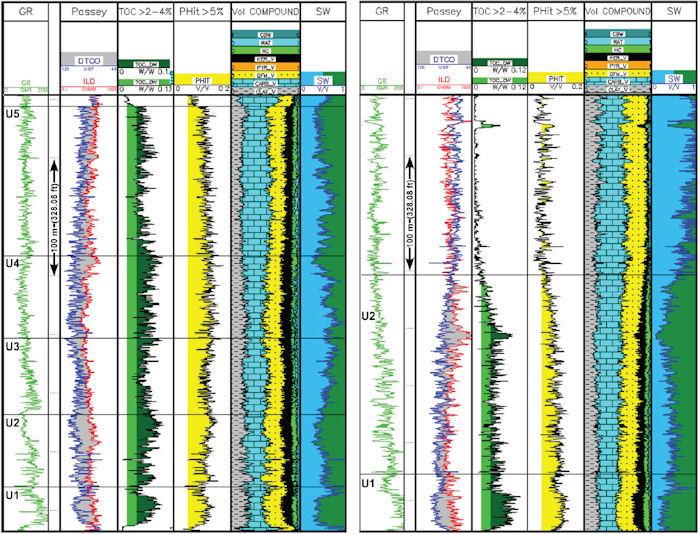
Geomechanics[edit]
The lesser the vertical heterogeneity and the lower the Young’s Modulus, the easier the fracture growth. Therefore, analyses indicate that the most appropriate geomechanical units to land the horizontal wells are the bottomsets of Unit 1 and Unit 2 that present numerous organic-rich mudstone beds with interfaces having weak geomechanical contrasts (homogenous vertical stack of lithofacies) and low Young’s Modulus (low stiffness allowing more aperture of the hydraulic fractures, hence more connectivity). Simulations estimate 30–50 m (98–164 ft) of fracture height in Unit 1[23].
The Vaca Muerta Formation has pressure gradients of 0.80–0.90 psi/ft. This over-pressure, caused by thermal maturation of the kerogen and the extremely low permeability of the rock.[4][24], enhances the mobility of the hydrocarbon and suggests to use more clusters per stage and more proppant in the hydraulic fracturing, and to reduce the importance of drilling on azimuth. Nevertheless, drilling on azimuth optimizes the production, as proved by experiments that show a 25%–50% increase of the EUR when the well azimuth is parallel to the present-day minimal horizontal stress (SHmin is north-south within a strike-slip stress regime)[25]. Note that the natural fracture network, coinciding with the present-day maximum horizontal stress (east–west), and the numerous bed-parallel veins (aka beefs) may be easily reactivated by the hydraulic fracturing, hence further increasing the Stimulated Rock Volume[26]. However, it is not yet proved that more natural fractures correlate with higher production and flow rates.
Organic Geochemistry[edit]
The organic material of Vaca Muerta, homogeneous in its composition within the basin, consists of amorphous material of aquatic marine origin, with scarce-to-null terrestrial contributions, and denotes excellent oil-prone quality. A century of activity of the O&G industry in the Neuquén Basin provided an extended database of tens of thousands of samples from cuttings, cores, sidewall cores, and outcrops of the Vaca Muerta Formation, from the entire basin. The evaluation of this data set identified six areas with different hydrocarbon potential (Figure 14). The areas broadly overlap with the morpho-structural domains (Figures 8, 12). The main resources encompassing high-quality low-sulfur oils and gas condensates are in the Embayment area, where stratigraphic units with high TOC are thick. The gases produced in other areas show a thermogenic marine origin (type II kerogen), with a lower contribution of biogenic methane[27] (1%–10%).
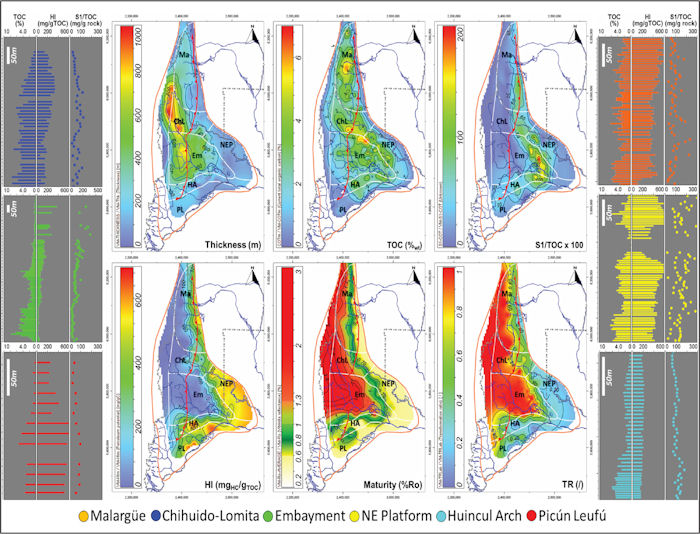
Basin Modeling[edit]
The onset of hydrocarbon generation from the Vaca Muerta occurred in the Albian in the western sector of the basin, in the Late Cretaceous in the Embayment area, and in the Tertiary in the northern area[28]. After the Mesozoic–Cenozoic burial, the exhumation of the stratigraphic column caused the Vaca Muerta source rocks to be out of equilibrium with the burial-related thermal maturity process, hence showing at present higher transformation and maturity parameters than those expected for the current depths. Part of the hydrocarbon generated from the Vaca Muerta was lost, part charged old structural highs, and part migrated to the basin margins through the underlying sandstones of Tordillo Formation. At the onset of the generation in the Embayment area (Figure 15), the uppermost part of Tordillo Formation lost the porosity because of cementation, preventing downward expulsion from Vaca Muerta[29]. This event, coupled with the pervasive generation within the thick section of low-permeability organic-rich mudstones, created an overpressured cell that retained most of the generated hydrocarbons within the source rock. This overpressured cell thinned out in fringe regions, allowing pressure dissipation and equilibration. In addition to the generation-related overpressure, recent uplift favored an increase of pressure disequilibrium by either low permeability transient effect or gas expansion[27].
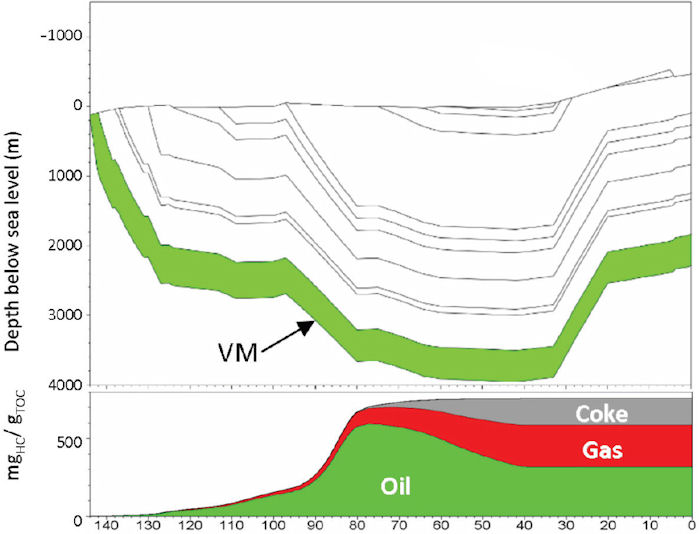
Play Concepts[edit]
The current play concept consists in landing the horizontal wells in the proximal bottomsets and lower foresets, which are the depositional sectors with the highest hydrocarbon storage potential and easiest fracture growth (i.e., larger stimulated rock volume). The rocks in these sectors show the best reservoir characteristics (TOC >2%, av. 5%; porosity >8%, av. 11%; clay <40%, av. 10–20%; water saturation <50%, av. 20%), the most adequate geomechanical properties (Young’s Modulus <4 Mpsi, low Poisson Ratio ~0.25, and homogeneous rock units exhibiting interfaces with weak geomechanical contrasts), and a thick vertical stack (av. 30–40 m) of lithofacies with the aforementioned characteristics (16A and window I and II in (Figure 16B). Although the distal bottomsets register the highest TOC, they are not a preferred landing zone because (with respect to proximal bottomsets) porosity is lower, clay is higher, ash beds have the highest recurrence, concretions and sills are more numerous, and landing zones are thinner ((Figure 16A). With this concept in mind, at least two landing zones have been fully de-risked and are producing in factory mode in the development phase (lower Unit 1 and lower Unit 2), and other six landing zones have been tested with production logging tool (PLT) data showing encouraging results (Figure 5). All current successful landing zones are in the Embayment area where hydrocarbon potential is the highest and wells produce high-quality low-sulfur oils and gas condensates.
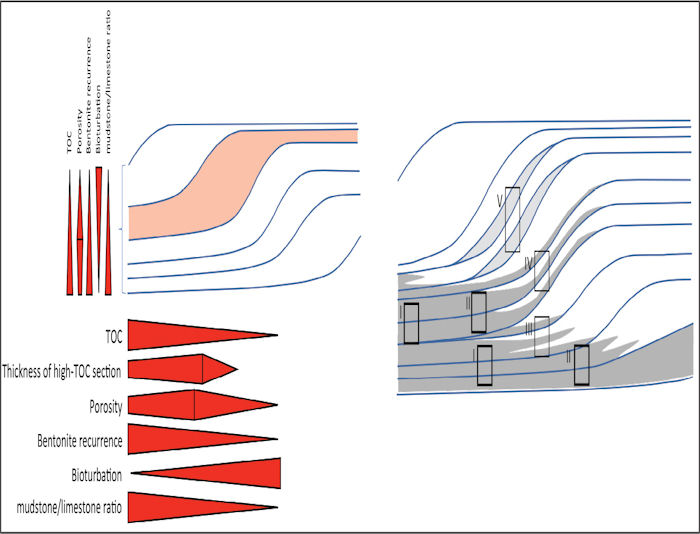
In the future, a further play concept may be tested in the foresets, where the number of landing wells has increased since 2019. As evidenced by subtle variations in seismic stacking patterns, the clinoforms did not prograde uniformly, and advanced (as a delta) with discrete steps, recording foresets with variable direction, extent, geometry, lithofacies, and degree of aggradational component. Within this variability, units with appropriate reservoir characteristics and thickness do exist in specific foresets, and represent promising prospects. This new play concept in the foresets consist of a stacking of thin foresets with good reservoir characteristics interbedded with thicker foresets with bad reservoir characteristics, creating a hybrid unconventional play, similar to the Niobrara play in the DJ Basin, the Bazhenov play in Russia, and the Wolfberry and Bone Spring plays in the Permian Basin ((Figure 16B, window III and V). Another option consists of transgressive units with good reservoir characteristics, typically deposited in the bottomsets, and cyclically deposited in the foresets. The interbedding of (1) muddy, high-TOC, transgressive units, climbing the clinoform from the bottomsets during sea level rise, and (2) coarse and lean foresets enhances the idea of a hybrid play where source rocks and tight reservoirs are interbedded ((Figure 16B, window IV). Furthermore, in the future, exploration may move beyond the Embayment area into the structural highs, in the fold-and-thrust belt, in sectors affected by igneous intrusions, and in areas where the Vaca Muerta is deeply buried.
Completion and Production[edit]
In the pilot phase, general industry practice consists in a plug and perf completion technique with: 1500–2000 m lateral drain, 15–20 fracture stages, 75–100 m of spacing between fracture stages, 3–5 clusters per stage, 900–1500 lb/ft (1339–2232 kg/m) of proppant, 1500–2000 m3 (52,972–70,629 ft3) of fluid per stage. Spacing between horizontal wells is approximately 300–400 m, a distance that mitigates frac hits and well interference but still drains most of the reservoir volume[5][23][30]. The geological understanding that defined the landing zones and the technological progress that introduced the multi-well pad drilling allowed operators to increase: (1) the lateral lengths from 500 to 3300 m (1500–10,000 ft), (2) the number of fracture stages from 3 to up to 54, (3) the amount of sand and fluids used, and (4) the geo-steering precision (from 10 m to 1 m) [6]. The cascading effect of the geological knowledge and technological progress was an increase in hydrocarbon production (Figure 4). In 2019, the average initial production gas rate was 400 km3/d (thousands of cubic meters per day) with 150 km3/d one year after being connected[23], and EUR was 200–300 million m3 (7–11 Bcf) of gas per well[30]. The average initial production oil rate was 600 boe/d, with 300 boe/d one year after being connected[5], and EUR was 1 Mbbl of oil per well.
A Final Thought[edit]
At present, the only profitable unconventional plays are in North America and Argentina. In Argentina, the Vaca Muerta play has continued to progress lowering the breakeven to US$40/bbl for oil and US$2.0 MMBtu for wet gas, and as activity ramps up these values will continue to fall making the operators believe that the combination of costs, well performance, and product prices are earning a return in their investments.
Unconventional potential exists in China, Saudi Arabia, Oman, Australia, Russia, Indonesia, Mexico, and United Kingdom, among others. These countries are considered to have rocks with the proven potential to deliver hydrocarbons from unconventional reservoirs, both self-sourced (shale gas and shale oil) and externally sourced (tight gas and liquid tight oil). The O&G industry is aware that these reservoirs differ in geological characteristics and require different technical approaches to enable commercial production (fair and predictable costs and prices aligned to the global market). However, the O&G industry also must consider the above-ground conditions (aka nontechnical risks) to guarantee safety and smooth operational workflows. The nontechnical risks are mainly related to mineral rights, water sourcing and disposal, infrastructure, regulations, surface use, as well as public opinion, geopolitical tensions, business terms, corruption, and crime. Altogether, the nontechnical risks will be key to determining whether these countries attract external investment to develop their unconventional potential. Some of the aforementioned countries, such as China, Australia, Russia, have tried, with mixed to poor success, to convert unconventional resources into economical reserves. However, the challenges outside North America are large to overcome. In fact, North America presents a legacy of 5 million wells that provide subsurface data, and has an extremely efficient, cost-competitive, and accessible supply chain to enable low-cost wells and infrastructure. Therefore, the economically successful development of unconventional resources outside of North America will require more than just good geology (as emphasized in section Conditions of economic success for Argentina). If the public opinion agrees, it will require enabling tax and royalty terms, an efficient regulatory framework, cost-competitive infrastructure, and a large enough supply chain to provide low-cost drilling and completion services. This takes time and a willingness to invest to realize.
In the Vaca Muerta play, it has taken O&G industry around 10 years and 1000 wells since the first hydraulically fractured wells in 2010 to get to the point where the wells are economically competitive with North America. It has taken 3–7 years for major O&G companies to become confident enough in the economics to sanction large developments. Nevertheless, with time, willingness to invest and good geology, the Unconventional Revolution will not remain a North American story, and the first areas to reach economically successful developments of unconventional resources will probably be the petroleum-rich provinces with important legacy data, substantial existing infrastructure, and public acceptance, previously described as Super Basins.
References[edit]
Other sources[edit]
- Veiga, R. D., G. D.Vergani, I. E.Brisson, C. E. Macellari, and H. A. Leanza, The Neuquén Super Basin, 2020: AAPG Bulletin, v. 104, no. 12, p. 2521–2555, doi: 10.1306/09092020023.
- ↑ Fryklund, R., and P. Stark, 2020, Super basins—New paradigm for oil and gas supply: AAPG Bulletin, v. 104, no. 12, p. 2507–2519.
- ↑ Howell, J. A., E. Schwarz, L. A. Spalletti, and G. D. Veiga, 2005, The Neuquén Basin: An overview, in J. Howell, E. Schwarz, L. A. Spalletti, and G. D. Veiga, eds., The Neuquén Basin, Argentina: A case study in sequence stratigraphy and basin dynamics: Geological Society (London) Special Publication 252, p. 1–14.
- ↑ Mosquera, A., and V. A. Ramos, 2006, Intraplate deformation in the Neuquén embayment, in S. A. Kay nad V. A. Ramos, eds., Evolution of an Andean Margin: A Tectonic and Magmatic View from the Andes to the Neuquén Basin (35°-39°S lat): Geological Society of America Special Paper 407, p. 97–123.
- ↑ 4.0 4.1 Notta R., E. Kruijs, V. Jain, G. Diaz-Perez, and H. Mandler, 2020, De-risking the Sierras Blancas and Cruz de Lorena blocks, black-oil window, in D. Minisini, M. Fantín, I. Lanusse Noguera, and H. A. Leanza, eds., Integrated geology of unconventionals: The case of the Vaca Muerta play, Argentina: AAPG Memoir 121, p. 445–468.
- ↑ 5.0 5.1 5.2 5.3 Vittore, F., D. T. Licitra, L. Monti, I. Lanusse Noguera, C. Hernández, H. Reijenstein, and J. Quiroga, 2020, Full development phase of the Loma Campana block: Black oil to gas and condensate windows, in D. Minisini, M. Fantín, I. Lanusse Noguera, and H. A. Leanza, eds., Integrated geology of unconventionals: The case of the Vaca Muerta play, Argentina: AAPG Memoir 121, p. 417–444.
- ↑ 6.0 6.1 6.2 Minisini, D., B. Fryklund, F. Gerali, and M. Fantín, 2020a, The first economical unconventional play outside North America: Context, history, and “coopetition”, in D. Minisini, M. Fantín, I. Lanusse Noguera, and H. A. Leanza, eds., Integrated geology of unconventionals: The case of the Vaca Muerta play, Argentina: AAPG Memoir 121, p. 1–24.
- ↑ Sattler, F., R. F. Domínguez, M. Fantín, P. Desjardins, H. Reijenstein, A. Bande, A. Liberman, O. Nielsen, M. Santiago, and D. Marchal, 2018, Enclosure 1 and 2, in González G., M. D. Vallejo, D. Kietzmann, D. Marchal, P. Desjardins, F. González Tomassini, L. Gómez Rivarola, and R. F. Domínguez, eds., Regional cross section of the Vaca Muerta Formation. Integration of seismic, well logs, cores and outcrops: Special Publication of Instituto Argentino del Petróleo y del Gas (IAPG), 244 p.
- ↑ U.S. EIA (Energy Information Administration) and V. Kuuskraa, 2011, World shale gas resources: An initial assessment of 14 regions outside the United States: U.S. Department of Energy, 365 p.
- ↑ Carmalt S. W., and A. Moscariello, 2017, What is a giant field?, ‘’in’’ R. K. Merrill and C. A. Sternbach, eds., Giant fields of the decade 2000–2010: AAPG Memoir 113, p. 9–14.
- ↑ González G., M. D. Vallejo, D. Kietzmann, D. Marchal, P. Desjardins, F. González Tomassini, L. Gómez Rivarola, and R. F. Domínguez, eds., 2018, Regional cross section of the Vaca Muerta Formation. Integration of seismic, well logs, cores and outcrops: Special Publication of Instituto Argentino del Petróleo y del Gas (IAPG), 244 p.
- ↑ WoodMackenzie, 2019, Argentina LNG exports positioned to meet peak demand in Asia: Wood Mackenzie, accessed May 2020.
- ↑ Ramos, V. A., V. Litvak, A. Folguera, and M. Spagnuolo, 2014, An Andean tectonic cycle: From crustal thickening to extension in a thin crust (34°–37° SL): Geoscience Frontiers, v. 5, p. 351–367.
- ↑ 13.0 13.1 13.2 Marchal, D., R. Manceda, R. F. Domínguez, and F. Sattler, 2020, [ https://archives.datapages.com/data/specpubs/memoir121/data/99_aapg-sp2120099.htm Structural geology: Tectonic history, macrostructures, regional fault map, fault systems, second-order structures, and impact of the inheritance], in D. Minisini, M. Fantín, I. Lanusse Noguera, and H. A. Leanza, eds., Integrated geology of unconventionals: The case of the Vaca Muerta play, Argentina: AAPG Memoir 121, p. 99–140.
- ↑ Stephenson, B., and Coflin, K., 2015, Guidelines for the handling of natural fractures and faults in hydraulic stimulated resource plays: SPE/CSUR Unconventional Resources Conference held in Calgary, Alberta, Canada, October 20–22, SPE-175910-MS.
- ↑ 15.0 15.1 Domínguez, R. F., H. A. Leanza, M. Fantín, D. Marchal, and E. Cristallini, 2020, Basin configuration during the Vaca Muerta times, in D. Minisini, M. Fantín, I. Lanusse Noguera, and H. A. Leanza, eds., Integrated geology of unconventionals: The case of the Vaca Muerta play, Argentina: AAPG Memoir 121, 141–163.
- ↑ Mitchum, R. M., and M. A. Uliana, 1982, Estratigrafía sísmica de las formaciones Loma Montosa, Quintuco y Vaca Muerta, Jurásico Superior y Cretácico Inferior de la Cuenca Neuquina, República Argentina: 1° Congreso Nacional de Hidrocarburos, Petróleo y Gas: Buenos Aires, Argentina, Actas, p. 439–484.
- ↑ Spalletti, L., and G. Veiga, 2007, Variability of continental depositional systems during lowstand sedimentation: An example from the Kimmeridgian of the Neuquén Basin, Argentina: Latin American Journal of Sedimentology and Basin Analysis, v. 14, p. 85–104.
- ↑ 18.0 18.1 18.2 Reijenstein, H. M., H. W. Posamentier, A. Bande, F. A. Lozano, R. F. Domínguez, R. Wilson, O. Catuneanu, and S. Galeazzi, 2020, Seismic geomorphology, depositional elements, and clinoform sedimentary processes: Impact on unconventional reservoir prediction, in D. Minisini, M. Fantín, I. Lanusse Noguera, and H. A. Leanza, eds., Integrated geology of unconventionals: The case of the Vaca Muerta play, Argentina: AAPG Memoir 121, p. 237–266.
- ↑ Minisini D., P. Desjardins, G. Otharán, M. Paz, D. Kietzmann, G. Eberli, C. Zavala, T. Simo, J. H. Macquaker, and C. Heine, 2020b, Sedimentology, depositional model, and implications for reservoir quality, in D. Minisini, M. Fantín, I. Lanusse Noguera, and H. A. Leanza, eds., Integrated geology of unconventionals: The case of the Vaca Muerta play, Argentina: AAPG Memoir 121, p. 201–236.
- ↑ 20.0 20.1 Kietzmann D. A., F. González Tomassini, and T. Smith, 2020, Grain association, petrography, and lithofacies, in D. Minisini, M. Fantín, I. Lanusse Noguera, and H. A. Leanza, eds., Integrated geology of unconventionals: The case of the Vaca Muerta play, Argentina: AAPG Memoir 121, p. 267–296.
- ↑ 21.0 21.1 21.2 21.3 Ortiz A. C., L. Crousse, C. Bernhardt, D. Vallejo, and L. Mosse, 2020, Reservoir properties: Mineralogy, porosity, and fluid types, in D. Minisini, M. Fantín, I. Lanusse Noguera, and H. A. Leanza, eds., Integrated geology of unconventionals: The case of the Vaca Muerta play, Argentina: AAPG Memoir 121, p. 329–350
- ↑ Cuervo, S., E. Lombardo, D. Vallejos, L. Crousse, C. Hernandez, and L. Mosse, 2016, Towards a simplified petrophysical model for the Vaca Muerta Formation: Unconventional Resources Technology Conference (URTeC), San Antonio, Texas, August 1–3, 19 p.
- ↑ 23.0 23.1 23.2 Estrada, S. J., M. F. Raverta, M. de Santa Coloma, J. P. Torres, and S. Galeazzi, 2020, Pilot phase of the Aguada Pichana Este block, Gas Window, in D. Minisini, M. Fantín, I. Lanusse Noguera, and H. A. Leanza, eds., Integrated geology of unconventionals: The case of the Vaca Muerta play, Argentina: AAPG Memoir 121, p. 497–514.
- ↑ Cuervo, S., E. Lombardo, and J. Adachi, 2018, Integration of 1D and 3D mechanical earth models in oil shale plays. An example from the Vaca Muerta Formation (Argentina): American Rock Mechanics Association, 52nd US Rock Mechanics/Geomechanics Symposium, Seattle, Washington, June 17–20, ARMA 18-856, v. 3, p. 1782–1791.
- ↑ Varela, R. A., D. Marchal, S. Cuervo, E. Lombardo, Y. S. Perl, D. E. Hryb, P. Pateti, and O. Nielsen, 2020, Geomechanics: Pressure, stress field, and hydraulic fractures, in D. Minisini, M. Fantín, I. Lanusse Noguera, and H. A. Leanza, eds., Integrated geology of unconventionals: The case of the Vaca Muerta play, Argentina: AAPG Memoir 121, p. 351–376.
- ↑ Ukar, E.,R. G. López, D. Hryb, J. F. W. Gale, R. Manceda, A. Fall, I. Brisson, E. Hernandez-Bilbao, R. J. Weger, D. A. Marchal, Z. Zanella, and P. R. Cobbold, 2020, Natural fractures: From core and outcrop observations to subsurface models, in D. Minisini, M. Fantín, I. Lanusse Noguera, and H. A. Leanza, eds., Integrated geology of unconventionals: The case of the Vaca Muerta play, Argentina: AAPG Memoir 121, p. 377–416.
- ↑ 27.0 27.1 27.2 27.3 Brisson, I. E., M. E. Fasola, and H. J. Villar, 2020, Organic geochemical patterns of the Vaca Muerta Formation, in D. Minisini, M. Fantín, I. Lanusse Noguera, and H. A. Leanza, eds., Integrated geology of unconventionals: The case of the Vaca Muerta play, Argentina: AAPG Memoir 121, p. 297–328.
- ↑ Brisson, I., 2015, Sistemas petroleros de la Cuenca Neuquina, in J. Ponce, A. Montagna, and N. Carmona, eds., Geología de la Cuenca Neuquina y sus Sistemas Petroleros: Viedma, Rio Negro, Argentina, Universidad Nacional de Rio Negro, p. 22–35.
- ↑ Veiga, R., F. Pángaro, and M. Fernández, 2002, Modelado bidimensional y migración de hidrocarburos en el ámbito de la dorsal de Huincul, Cuenca Neuquina-Argentina: 5° Congreso de Exploración y Desarrollo de Hidrocarburos, Mar del Plata, Argentina, 29 October–2 November, p. 1–22.
- ↑ 30.0 30.1 Biscayart, P., M. Brolli, J. d’Hiriart, D. García Acebal, A. Giachino, S. Olmos, N. Requena, and R. Varela, 2020, “Factory mode” development of Fortín de Piedra block, gas window, in D. Minisini, M. Fantín, I. Lanusse Noguera, and H. A. Leanza, eds., Integrated geology of unconventionals: The case of the Vaca Muerta play, Argentina: AAPG Memoir 121, p. 515–528.